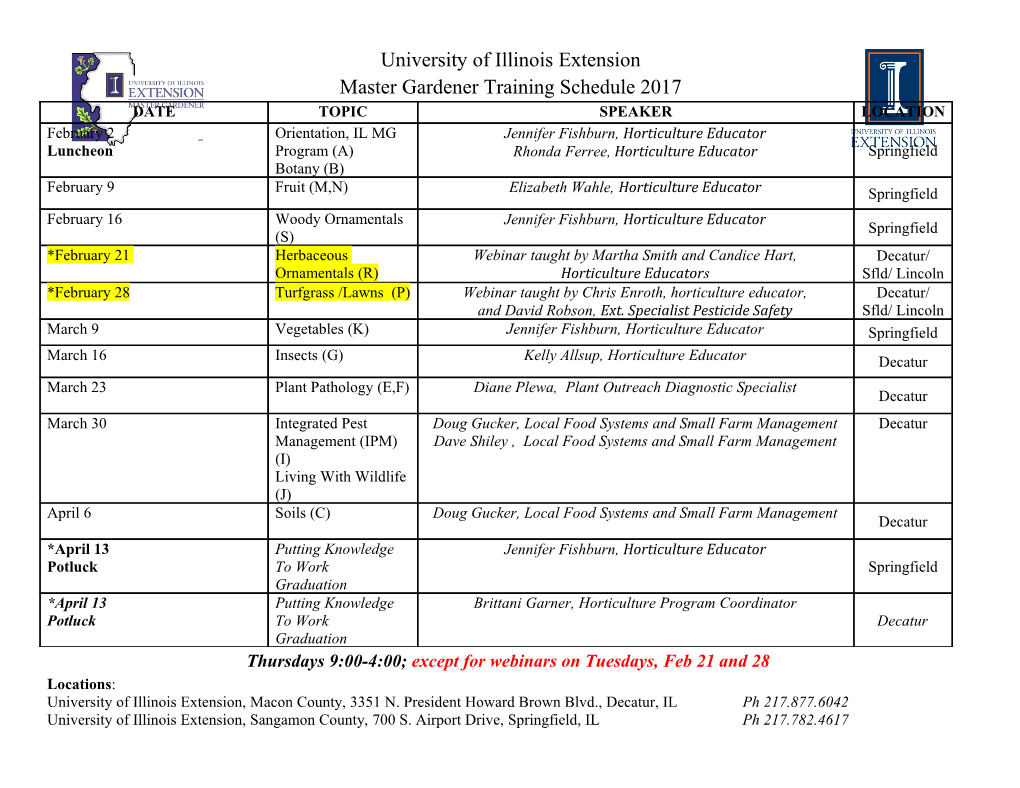
Planetary Science Vision 2050 Workshop 2017 (LPI Contrib. No. 1989) 8071.pdf Getting humans to Mars, a possible future. S. Diniega1, D. Beaty1, D. Bass1, L. Hays1, C. Whetsel1, R. Whitley2, R. Zurek1, 1Mars Program Office, Jet Propulsion Laboratory, California Institute of Technology, 4800 Oak Grove Dr., Pasadena, CA 91001 USA ([email protected]), 2NASA Johnson Space Center, Houston, TX 77058. The context: We envision that it is 2050 and humans the surface, monitoring of the atmosphere, and tele- are exploring the Martian surface in situ. They survive communications with the surface crew. (This increase on Mars for over a year at a time, with crews rotating in monitoring capability also yielded important science through. Over a series of missions, a habitat and sever- results in development of surface and atmospheric pro- al support building have been constructed. Within the cesses and transport models, as measurements and im- latter, crops are grown and resources like fuel are gen- aging were concurrently acquired over a range of loca- erated, decreasing the amount of basic supplies brought tions and times-of-day. Numerous, small payloads also in from Earth and opening missions’ “delivery” vol- got to Mars, riding with the primary missions.) umes to more interesting tools and more complicated These images were paired with high resolution resources. Although water sufficient for current needs spectral maps of the surface and near-surface radar is being sourced from the local region, a second habitat scans (<10m and 10s-m planform resolution, respec- is being planned at higher latitudes where subsurface tively) – allowing for careful matching between the ice is more easily accessible – at least during the warm high-resolution (sub-meter resolution) images/DTMs seasons. Support staff monitors conditions at the sur- and maps of subsurface structure and surface composi- face and within the atmosphere 24-7 (via visible image- tion (which also feed into 2. Bio. & Geochem. Recon.). ry, atmospheric profiles, and regular communications Although these datasets have larger footprints than the with the Mars surface crew), allowing for weather fore- high-resolution imagers and don’t require overlapping casts and early storm warning systems, which is espe- acquisition, it still took decades to build up the desired cially important during the dust storm season. Repeat coverage at the sufficient resolution for resource map- high resolution imaging, coupled with spectral imaging ping and development of mining/processing plans and radar data, allows for careful geologic mapping of (which, again, is needed for picking the landing site). portions of Mars likely to contain resources and/or Additionally, as only a handful of locations have been hazards. And detailed laboratory experiments, con- sampled in situ and those only to a shallow depth (10s ducted on the martian surface and with martian samples of centimeters), decades of work were needed (and delivered to Earth laboratories, have been and are an- continues to be needed) for the creation and refinement swering key questions about biological potential and of 3D geologic maps from orbital data, as well as de- geologic history of surface materials – which feed into velopment of surface/near-surface process models that high-priority science as well as decisions about ISRU feed into identification, mapping, and characterization design and Planetary Protection (PP) protocols. of resource reservoirs (e.g., accessible water ice, hy- Datasets needed to enable this exploration [1-3]: drated minerals and/or propellant reactants within a 1. Orbital Infrastructure, Observations, and Telecom- sufficiently extensive, near-surface geologic unit). munications. To support the humans and their neces- These resources are varied in type and sometimes are sary infrastructure (which includes picking the landing co-located with hazardous materials (avoidance of sites), a systemic collection of high-resolution images which requires at least detection). and measurements were needed. Such imagery has Atmospheric measurement and monitoring has also been collected over decades as the high-resolution im- been continued via a few orbital missions over the last ages have small footprints and in many cases repeat few decades, extending the timeline begun with the images are needed – to monitor changes, to construct MCS and MARCI in 2006. Near-continuous monitor- digital terrain models (DTMs) from stereo image pairs, ing with coarse resolution, global images (showing and to understand the landscape under different light- e.g., cloud cover and dust storm development) and ing and seasonal conditions. For comparison, the atmospheric profiles of volatiles, dust/aerosol content, HiRISE camera on MRO collected images covering and temperature over this long temporal baseline has ~2% of Mars over a decade; with advances in downlink allowed for atmosphere models to be developed and capabilities and onboard storage, the rate of image ac- refined to the level used within terrestrial climate mod- quisition was increased by a factor of at least 3 with the eling – which is a crucial input for creating the context following orbiter. However, a substantial increase in for weather forecasting and for enabling more precise image acquisition didn’t occur until construction of the and larger-mass entry, descent, and landing (EDL) communications and reconnaissance network of satel- technology. During the last decade, network reconnais- lites was begun, allowing for uninterrupted imaging of sance satellites were also able to measure near-surface winds, which coupled with proxy measurements of Pre-decisional: for information and discussion only Planetary Science Vision 2050 Workshop 2017 (LPI Contrib. No. 1989) 8071.pdf upper-atmosphere wind flow (e.g., cloud movement) sultant improvements in the understanding of the pre- allows for important refinement of the martian climate sent-day martian surface conditions and history of the and weather models and improved interpretation of evolution of these materials has provided a fuller set of geologic climate records. contextual information to planners of min- 2. Biological and Geochemical Reconnaissance. A ing/processing, construction, and operations processes. high-priority piece of information for both science and Missions needed to generate these datasets, in this human exploration advancement was whether or not possible future [1-3]: Mars has extant life, and if so it is everywhere (includ- Several Mars orbiters have been needed over the ing in the airborned dust) or if it is restricted to refu- years, first to replenish imaging capabilities (MRO, gia. This needed to be known in order to design the PP the Next Mars Orbiter [7], and those that followed – aspects of the human landed mission. In particular, at least one per decade), then within the telecom- we’d needed to know if Mars has extant life as that is munications satellite network that incorporated nec- an important influence on how humans will begin in- essary visible and spectral imaging capabilities, teracting with that life and its habitat. One critical ena- greatly increasing coverage and allowing for con- bling data set of this was the mapping of “Special Re- current monitoring over multiple locales. Also in- gions” [4] (see 1. Orbital Infrastr., Obs., & Telecom.), corporated within these orbiters were atmosphere which allowed these potential habitable regions to be and radar instruments that allowed for the needed planned around and carefully investigated via orbital atmospheric reconnaissance (e.g., wind, tempera- and landed robotic missions. A second critical enabling ture, and aerosol profiles) and biological and geo- data set was the study of samples of martian regolith chemical reconnaissance for the identification and that were returned to Earth. Additionally, from the characterization of sub-surface resource reservoirs returned sample studies, as well as in situ measure- and for PP assessments. ments, it was determined whether the regolith and/or Mars Sample Return (MSR) enabled careful meas- airfall dust contained potentially hazardous concentra- urement of surface material properties and compo- tions of certain “poisonous” compounds (such as CrVI) sition, yielding information about e.g, machine and so as to engineer around them. human hazards (e.g. dust size and amount, toxicity). 3. ISRU Exploration. As we intended to set up a base Such information was vital for the design of habitat, that was revisited, it was necessary to invest in the ex- transport, and ISRU processes and machinery. ploration for water deposits. This began with orbital A progression of more complicated and larger-scale recon (see 1. Orbital Infrastr., Obs., & Telecom. and 2. landed missions focused on ISRU technology Bio. & Geochem. Recon.), but that alone was not demonstrations were needed, before full-scale enough. At least one exploration mission to the martian ISRU mining and processing could commence and surface was also required to define what on Earth are enough of the critical resources could be produced called “reserves”: deposits for which all of the essential on Mars for stable human habitation. This involved attributes have been defined, such that a known min- much iteration between the reconnaissance and ing/processing system can interact with it with predict- characterization of the martian environment, and ible results [5]. development of the machinery and processes. 4. ISRU Engineering. A critical antecedent to large-
Details
-
File Typepdf
-
Upload Time-
-
Content LanguagesEnglish
-
Upload UserAnonymous/Not logged-in
-
File Pages2 Page
-
File Size-