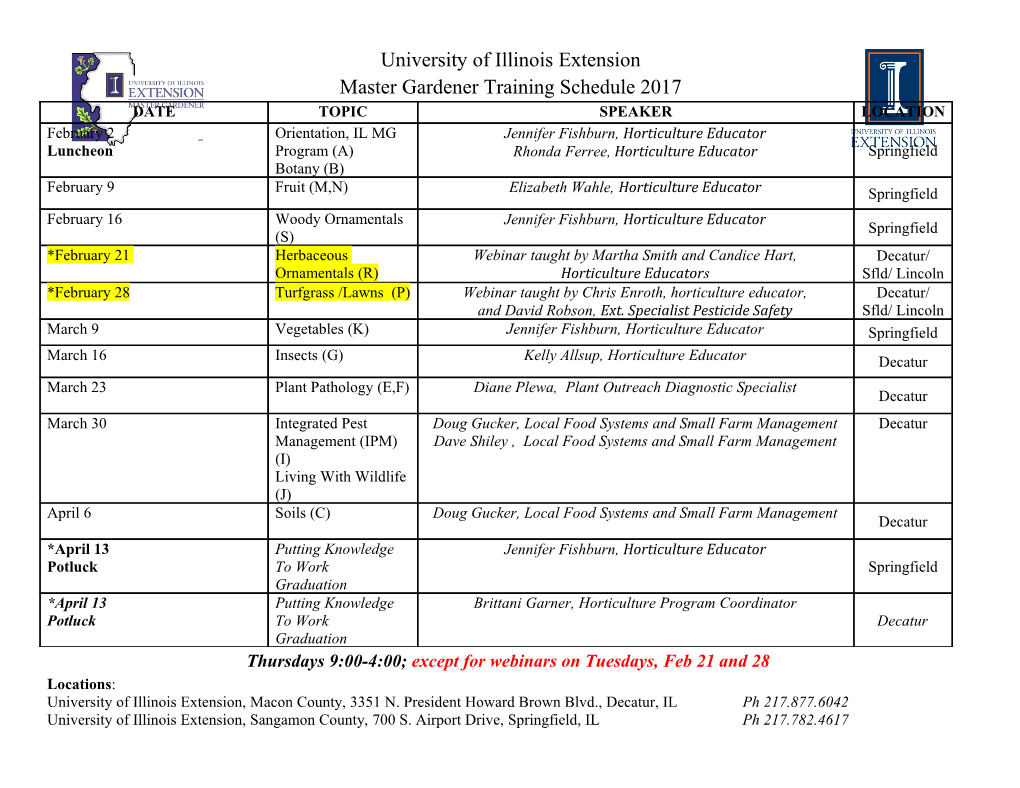
MICROBIOLOGICAL REVIEWS, Sept. 1984, p. 181-198 Vol. 48, No. 3 0146-0749/84/030181-18$02.00/0 Copyright © 1984, American Society for Microbiology Bacterial Triterpenoids RICHARD F. TAYLOR BioMedical Research and Technology Section, Arthur D. Little, Inc., Acorn Park, Cambridge, Massachusetts 02140 INTRODUCTION ............................................................ 181 TRITERPENOID BIOSYNTHESIS ........................................................... 181 BACTERIAL TRITERPENOIDS: OCCURRENCE ............................................. 182 Acyclic Triterpenoids ........................................................... 182 Hopanoids and Sterols ........................................................... 186 EVOLUTION AND BIOSYNTHESIS OF BACTERIAL TRITERPENOIDS .... .................. 188 FUNCTION OF BACTERIAL TRITERPENOIDS .......... .................................. 190 Membrane Structure ............................................................ 190 Other Functions ............................................................ 193 CONCLUSION ........... ............................................... 194 LITERATURE CITED ............................................................ 194 INTRODUCTION precursor has been identified, it has been found to be squalene. Triterpenoids and their precursor isoprenoid hydrocar- In the second major pathway of terpenoid formation, two bons are among the oldest known and most ubiquitous molecules of geranylgeranyl pyrophosphate (C20) are dimer- chemicals on the earth, having been found in fossil remains ized to form the first, acyclic, parent tetraterpenoid (C40), from a variety of geological formations and sediments (45, which then serves as the precursor for all other tetraterpen- 47, 81, 101, 105, 108). The reconstruction of biosynthetic oids and tetraterpenoid derivatives. In all likelihood, this pathways from such records indicates that the ability to first acyclic tetraterpenoid is lycopersane, although ques- synthesize isoprenoids such as pristane and phytane and, tions still remain as to whether it is phytoene (32). later, triterpenoids such as squalene and hopanes was pres- Of the two higher terpenoid classes, the triterpenoids ent in non-photosynthetic bacteria before the evolution of contain the greatest variety of unique biosynthetic end chlorophyll biosynthesis. The occurrence of triterpenoids in products. These include acyclic derivatives resulting from bacteria, and especially in non-photosynthetic bacteria, is either desaturation (triterpenoid carotenoids) or reduction thus of interest from both evolutionary and functional as- (reduced squalenes) of the parent triterpenoid and cyclic pects. This interest has been further stimulated by the derivatives such as the hopanoids and sterols. Although discovery and identification of a number of unique triterpen- some exceptions do occur, such as the occurrence of the oids in bacteria during the last decade and by conclusive pentacyclic triterpenoid tetrahymanol in the protozoan Tet- proof that sterols can occur in procaryotes. rahymena pyriformis (82), exclusive domains for various The purpose of this review is to present an overview of triterpenoids appear to exist. For example, with the excep- triterpenoid occurrence and function in bacteria. To accom- tion of squalene and the immediate biosynthetic precursors plish this goal, the two major classes of known bacterial of the cyclic triterpenoids (such as squalene-2,3-epoxide), triterpenoids, i.e., acyclic and cyclic, are reviewed. Particu- the acyclic triterpenoids have been found exclusively in lar emphasis is focused on the acyclic triterpenoid carot- bacteria. Of the cyclic triterpenoids, pentacyclic compounds enoids and the cyclic hopanoids since these two groups of represent the major group found in bacteria. As discussed compounds are rapidly emerging as major classes of bacteri- later, sterols, although present in some bacteria, are not a al natural products. This review does not address blue-green major product of bacterial triterpenoid biosynthesis. Al- algal (cyanobacterial) triterpenoids since this subject has though the natural occurrence of mono- and dicyclic triter- been addressed elsewhere (100, 101), nor are the general penoids has been reported (98, 100), neither class is yet topics of microbial uptake and metabolism of sterols dis- known in bacteria. cussed except in those cases where these events involve a The tetraterpenoids, although not as structurally diverse sterol synthesized de novo by the bacterium. as the triterpenoids, nevertheless represent a major natural product class, ubiquitous throughout the plant and animal TRITERPENOID BIOSYNTHESIS kingdoms. Tetraterpenoids are represented exclusively by Before discussion of bacterial triterpenoids, a general the tetraterpenoid carotenoids (C40-carotenoids) and their description of their place in terpenoid biosynthesis is re- derivatives. Another group of tetraterpenoids has been re- quired. Figure 1 presents a general scheme of higher terpe- ported, i.e., the C40-w,w'-biphytanyl diols of thermoacido- noid formation (i.e., containing six or more isoprenoid [C5] philic bacteria (29, 35, 36, 76, 165). The biosynthesis of these units and synthesized by head-to-head condensation of ap- compounds does not appear, however, to occur via geranyl- propriate C15 or C20 isoprenoid precursors). Basically, two geranyl pyrophosphate condensation, but rather by a bridg- pathways are known. In the first, two molecules of farnesyl ing reaction between the two O-phytanyl side chains of a di- pyrophosphate (C15) undergo reductive dimerization (after O-phytanyl glycerol molecule. allylic rearrangement of one to nerolidol pyrophosphate) to Only microbes and plants are known to synthesize the C40- form the first, acyclic, parent triterpenoid (C30). Other carotenoids. Animals (as well as microbes and plants) can, acyclic and the cyclic triterpenoids are then derived from the however, oxidatively degrade C40-carotenoids to "apo-ca- initial condensation product. In all cases where the first C30 rotenoids" and can utilize the resulting products as essential 181 182 TAYLOR MICROBIOL. REV. C15 + C15 C20+ C20 C30 C40 TETRATERPENOID SATURATION DESATURATION CAROTENOIDS CYCLIZATION (MICROBES, PLANTS) DIHYDROSQUALENES | TRITERPENOID (BACTERIA) CAROTENOIDS + Cs (BACTERIA) C45; C50 <C40 I --I HOMO-CAROTENOIDS APO-CAROTENOIDS PENTACYCLIC TETRACYCLIC STEROLS (BACTERIA) (MICROBES, PLANTS, ANIMALS) TRITERPENOIDS TRITERPENOIDS (MICROBES, PLANTS, (MICROBES, PLANTS) (PLANTS, ANIMALS) ANIMALS) FIG. 1. End products of terpenoid biosynthesis. In general, acyclic triterpenoids other than squalene are found only in bacteria. Cyclized triterpenoids are found throughout the plant and animal kingdoms. Tetraterpenoids are represented exclusively by carotenoids and their derivatives. nutrients, e.g., as sources of vitamin A. The biosynthesis of found to contain both sterols/hopanoids and C30-carot- C45- and C50-carotenoids (the "homo-carotenoids") is enoids, an evolutionary trait which is discussed later. Only known to occur only in non-photosynthetic bacteria (169) one organism, Methylococcus capsulatus, has been found to and involves addition of C5-isoprenoid units onto one or both contain both hopanoids and sterols. ends of a C40-carotenoid. End-terminal cyclization of C40- The amounts of triterpenoids occurring in bacteria vary carotenoids (an event yet unknown for C30-carotenoids) can from 0.0001 (the practical detection limit) to -0.6% by be carried out by both microbes and plants to result in either weight (i.e., 1 to 6,000 ,ug/g, dry weight). In order of lowest mono- or bicyclic products (17). The formation of more to highest content found to date, these are as follows: highly cyclized tetraterpenoids is not known to occur. diapophytoene (0.02%), total C30-carotenoids (0.05%), re- duced squalenes (0.2%), sterols (0.2%), squalene (0.4%), and BACTERIAL TRITERPENOIDS: OCCURRENCE hopanoids (0.6%). Table 1 summarizes the known occurrence of triterpen- Preliminary claims have also been made that hopanoids oids in bacteria. In using this table, however, it should be exist in a variety of other bacteria including species of noted that in some cases the exact taxonomy of a bacterium Rhodopseudomonas, Methylomonas, Hyphomicrobium, is subject to further study and potential change. For exam- and Nitrosomonas (108, 119). These organisms are not listed ple, Sarcina litoralis may be more accurately classified as a in Table 1 since no data have yet been published identifying Halococcus sp. (22, 68) and is placed in the table according- the hopanoids present. It is notable, however, that all of the ly. Amoebobacter morrhuae has been tentatively reclassi- bacteria reported by these authors as having hopanoids have fied as a Halobacterium sp. (22), and the exact identity of G+C contents of 50 to 73 mol% and include aerobic and Pseudomonas rhodos is unclear (and its guanine-plus-cyto- facultative anaerobic chemoorganotrophs, chemolitho- sine [G+C] content has not been reported). In addition, as trophs, and photoorganotrophs. Also, a number of bacteria with any evolving study area, additional bacterial triterpen- are reported as not containing hopanoids. These include a oids will undoubtedly be discovered and potentially change number of bacteria with G+C contents of <50 mol%, i.e., the apparent trends discussed in this review. Streptococcus faecium, Proteus vulgaris, Bacillus subtilis, With such limitations in mind, a number ofgeneral conclu- Clostridium paraputrificum,
Details
-
File Typepdf
-
Upload Time-
-
Content LanguagesEnglish
-
Upload UserAnonymous/Not logged-in
-
File Pages18 Page
-
File Size-