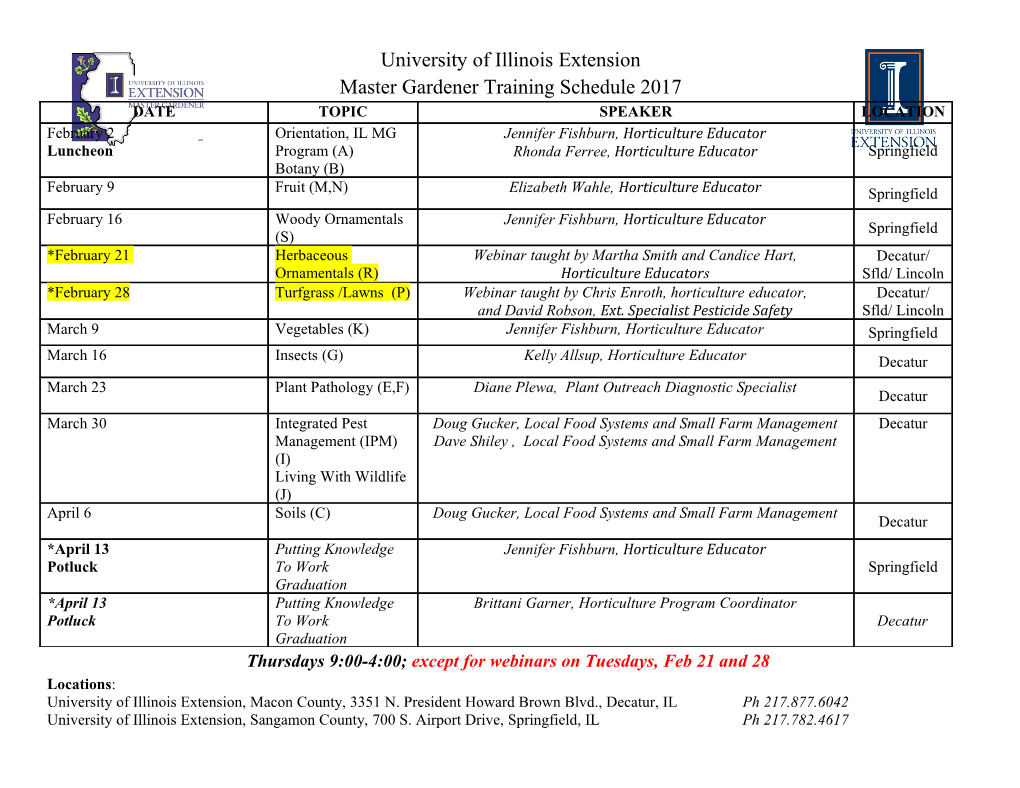
Role of Albumin in Hepatic Stellate Cell Activation The Antifibrotic Effects of Albumin Master’s Research Report 1 Author: B.W. Perridon. S2031582. Master’s student in Biomedical Sciences at University of Groningen. Internship: December 2013 – July 2014. Report: May 6, 2015. Department of Gastroenterology and Hepatology, University Medical Center Groningen. Supervisors: prof. dr. K.N. Faber and F.W. Haijer. Abstract Introduction Liver fibrosis and cirrhosis are caused by a continuous wound healing response of the liver, characterized by scar formation and the deposition of excessive amounts of extracellular matrix components by hepatic myofibroblasts. Hepatic stellate cells (HSCs) are currently considered as the major source of the hepatic myofibroblasts. During fibrogenesis the role of the HSCs in the liver changes as they undergo a phenotypic transformation from their quiescent to an activated, myofibroblast-like phenotype. In pancreatic stellate cells, cells that are comparable to HSCs, it was suggested that albumin has a direct effect on (pancreatic) stellate cells activation. Whether albumin is produced by HSCs or whether they acquire it from extracellular sources is unknown, as well as its potential role in HSCs. Aims of the study were to investigate the presence and the source of albumin in HSCs and its effect on the activation of HSCs. Methods Primary HSCs, portal myofibroblasts, hepatocytes and Kupffer cells were isolated from male Wistar rats. The presence of albumin protein in HSCs cultured in different conditions was examined using Ponceau S staining and Western blotting. Immunofluorescence microscopy was used to study the intracellular localization of albumin. The production of albumin by HSCs and the expression of quiescent HSC markers (PPAR γ; LRAT) and HSC activation markers ( αSMA; collagen type 1a1) were studied using qPCR. Potential contamination of HSCs by hepatocytes was analyzed using hepatocyte markers (BAAT; BSEP). Uptake of albumin orthologs by HSCs was examined using Western blotting. Effects of albumin administration to culture-activated HSCs on the HSC activation markers were determined using qPCR. Results Western blotting and immunofluorescence microscopy revealed the presence and location of albumin in both quiescent and activated HSCs. Albumin mRNA levels decreased upon culture-activation of HSCs in a similar fashion as the hepatocyte markers BAAT and BSEP, suggesting that it was (virtually) absent in both HSC phenotypes. Primary rat HSCs only contained rat albumin when cultured in rat serum containing medium but contained bovine/calf albumin when cultured in fetal calf serum containing medium. Bovine/calf albumin dose-dependently reduced the expression of the HSC activation markers collagen type 1a1 and αSMA when added to the culture medium at concentrations above 22.5 g/L, which is important since the normal blood level of albumin ranges from 35 to 55 g/L and is thus markedly higher than the albumin concentration normally used in culture. Conclusion These data show that HSCs do not produce albumin but that they do actively take up albumin from extracellular sources, suppressing HSC activation in a concentration and time dependent manner. Based on the finding that albumin has antifibrotic properties, we propose a mechanism in which healthy hepatocytes attenuate liver fibrosis through albumin. Table of contents Abstract ................................................................................................................................................... 1 Introduction .............................................................................................................................................. 3 Materials and methods ............................................................................................................................. 6 Animals ............................................................................................................................................... 6 Cell isolation and culture ...................................................................................................................... 6 Experimental design ............................................................................................................................ 7 Protein isolation ................................................................................................................................... 8 RNA isolation and cDNA synthesis ...................................................................................................... 8 Real-time quantitative PCR .................................................................................................................. 9 Western blot analysis ........................................................................................................................... 9 Immunofluorescence microscopy ....................................................................................................... 10 Statistical analysis ............................................................................................................................. 10 Results .................................................................................................................................................. 10 Albumin present in both quiescent and activated HSCs ...................................................................... 10 Albumin not expressed by HSCs ........................................................................................................ 12 Albumin taken up by HSCs ................................................................................................................ 16 HSC activation delayed by albumin .................................................................................................... 16 HSC activation not only controlled by albumin .................................................................................... 18 Conclusions and discussion ................................................................................................................... 19 Bibliography ........................................................................................................................................... 23 Supplemental data ................................................................................................................................. 27 Abbreviations: aHSC: activated hepatic stellate cell; αSMA: alpha-smooth muscle actin; BAAT: bile acid- CoA:amino acid N-acyltransferase; BSA: bovine serum albumin; BSEP: bile salt export pump; col-1: collagen type 1a1; ECM: extracellular matrix; FCS: fetal calf serum; HSC: hepatic stellate cell; LRAT: lecithin retinol acyltransferase; PMF: portal myofibroblast; PPAR γ: peroxisome proliferator activated receptor γ; PSC: pancreatic stellate cell; qHSC: quiescent hepatic stellate cell; RS: rat serum; TGF-β: transforming growth factor β. 2 Introduction The liver is a vital organ that plays a major role in a great variety of metabolic processes. It is involved in storage, synthesis and breakdown of small and complex molecules such as proteins, carbohydrates and lipids. The liver is also involved in clearance of potentially harmful compounds. The organ can deal with damage in a way no other organ can as it can completely regenerate from a small portion of healthy liver. The regenerated liver tissue (partly) reverses the loss of liver function caused by the damage. All populations of cells within the liver are able to regenerate and migrate to the regenerated tissue [Reviewed by [1] . In case of repetitive damage, in for example chronic liver diseases, the liver becomes fibrotic as it attempts to replace and repair damaged tissue. Apoptotic hepatocytes, Kupffer cells and inflammatory cells greatly contribute to this process. The hepatic tissue repair reaction is characterized by the formation of highly dynamic scar tissue and a shifted balance between the production and degradation of connective tissue. This results in the deposition of excessive amounts of extracellular matrix (ECM) components, mainly fibrillar collagen, by hepatic myofibroblasts [2, 3] . It has been speculated that hepatic myofibroblasts, normally absent in the liver, are able to promote liver regeneration of the damaged liver by producing growth factors for hepatic oval cells [4] and probably also for other cells involved in the regeneration. However, it is generally accepted that an accumulation of hepatic myofibroblasts causes fibrosis and can result in a progressive loss of liver function called cirrhosis. There is increasing evidence that hepatic fibrosis is reversible if the stimuli are successfully removed [5, 6] . Eventually liver fibrosis can progress to liver cirrhosis. In general perpetuation to cirrhosis takes months to years of liver disease. Cirrhosis is characterized by a loss of liver architecture that results from excessive fibrosis and the formation of pseudolobules, also called regeneration nodules, throughout the liver [Reviewed by [7] . The process of cirrhosis causes liver dysfunction and distorts the fundamental hepatic architecture making reversal more difficult. Liver and its cell types The complex functions of the liver mentioned above are mainly performed by hepatocytes, also known as hepatic parenchymal cells. Hepatocytes are orchestrated in liver lobules, which are small functional units of the liver. Besides hepatocytes, liver lobules contain non-parenchymal cells like the
Details
-
File Typepdf
-
Upload Time-
-
Content LanguagesEnglish
-
Upload UserAnonymous/Not logged-in
-
File Pages30 Page
-
File Size-