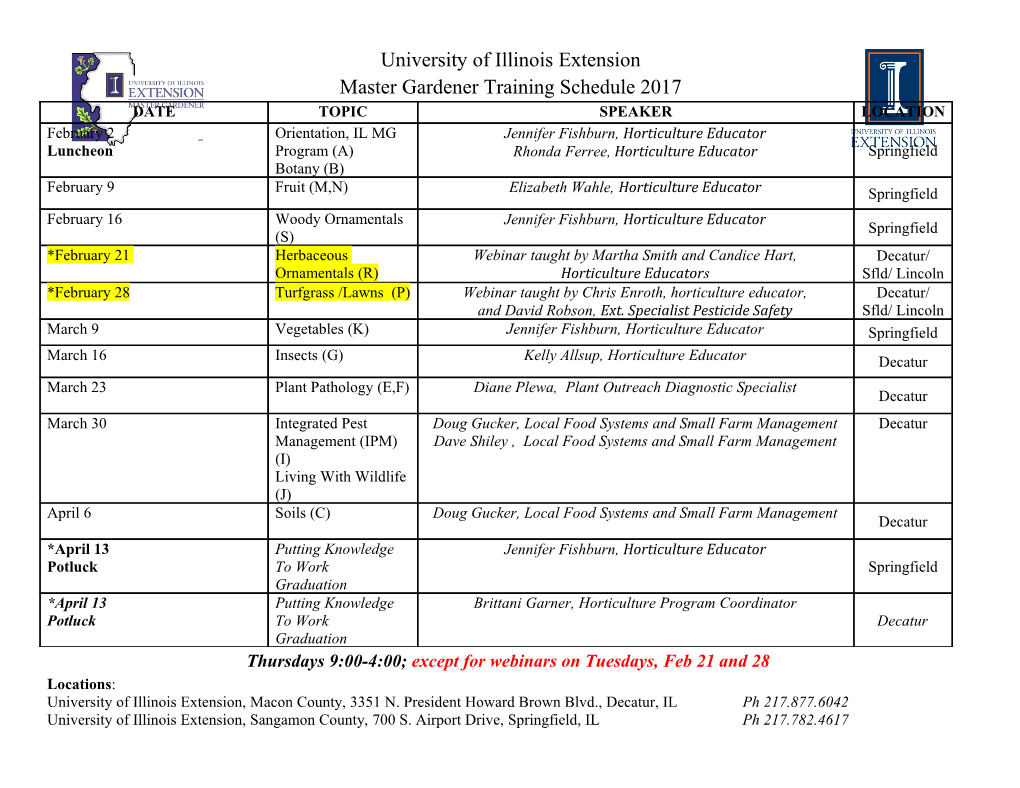
A Review on the Management of E-waste and the Role of Urban Mining: A Global Assessment By Jacob Bowden Mentors: Dr. Raymond Farinato, Professor of Professional Practice, Earth & Environmental Engineering, Columbia University Dr. R. Nagaraj, Professor of Professional Practice, Earth & Environmental Engineering, Columbia University Dr. Athanasios Bourtsalas, Lecturer, Energy and Materials, Earth & Environmental Engineering, Ass. Director, Circular Economy, Earth Engineering Center, Columbia University EXECUTIVE SUMMARY The rapid advancement of technology over the past few decades has undoubtedly benefitted society in countless ways. Consumers benefit from more advanced, faster, and more reliable technology, which has created new opportunities in health, education, government, entertainment, and business. However, the rapid development of these new technologies entices consumers to purchase newer and more advanced products, and thus, electronics quickly become obsolete in a “new is better” society. Unfortunately, the rapid growth in electronics has not been met with proportionate growth in the collection, reuse, or recycling of those products as they reach end-of-life (EOL), thus resulting in an exponential increase in e-waste generation world-wide. It is now the fastest growing form of waste worldwide, increasing at a rate of 3 to 5% every year. Globally, it is expected that 52 million tons of e-waste will be discarded in 2021. Asia generates the largest amount of e-waste, producing over 40% of the globe’s total volume, but it only collects and recycles 15% of that waste (Balde et al., 2017). Solving this problem requires an integrated approach involving multiple key players including government, business, consumers, and formal recycling companies. This paper focusses on one aspect of the solution to e-waste: urban mining. Despite the fact that e-waste only makes up two percent of the world’s waste in landfills, it comprises 70 percent of all heavy metals and causes enormous damage to both the environment and human health, especially in developing countries where most of it is disposed of improperly (Jiang et al., 2012). Approximately 80% of all e-waste that is collected in developed countries is exported to developing countries such as China, India, Nigeria, and Ghana (Solving the E-waste Problem, 2009). These countries lack the regulation and infrastructure to dispose of e-waste safely, and hence, primitive recycling methods cause significant harm to the environment polluting the soil, air, and aquatic ecosystems. E-waste recycling sites also adversely affect human health as over 1,000 toxic substances found in e-waste harm the human body, causing lead and other heavy metal poisoning, cancers, kidney disease, neurotoxicity, liver damage, fetal toxicity, and skin and eye irritations, among others. Although e-waste is categorized as hazardous waste, it also contains precious metals such as gold, silver, platinum, and palladium and other valuable materials, 90% of which can be recycled and reused in new electronic devices (Prasad et al., 2020). The term urban mining describes the process of recovering precious metals and energy from e-waste streams through sustainable recycling methods. It consists of three main phases: collection, pre-processing, and end-processing. Collection is crucial because it determines the amount of material available for recovery. During pre-processing, products are dismantled, materials are separated from each other, and hazardous substances are removed. End-processing options may include pyrometallurgical, hydrometallurgical, and biometallurgical methods to recover and purify copper, gold, silver, and palladium and other valuable materials. Three major global smelters are the Boliden Rönnskär Smelter in Sweden, the Umicore Precious Metals Refining plant in Belgium, and the Horne Smelter in Canada. Urban mining plays a key role in the effort to reduce and valorize the world’s rapidly increasing e- waste. Proper urban mining programs can have positive environmental, health, and economic impacts, which include: 1. releasing fewer harmful elements and generating less CO2 emissions than primary mining; 2. reducing the need for new resources and preserving rare earth elements that are effectively non-renewable; 3. reducing the amount of e-waste directly deposited into landfills and the amount of toxins released into the environment through primitive recycling methods, and in turn, reducing human exposure to these toxins; 4. generating massive financial potential as The United Nations University estimates the current amount of global e-waste is worth 55 billion euros of raw materials (Balde et al., 2017); 5. using significantly less energy than primary mining since e-waste streams hold a much higher concentration of valuable minerals, and “The proportion of valuable metals that can be recovered from e-waste is up to ten times greater than the amount extracted from primary mineral deposits” (Xavier, 2019); 6. reducing countries’ dependency on China for rare earth elements (REE) which holds about 90% of the world’s supply of REE (Xavier, 2019); 7. helping to create circular economies to recover the precious and special metals required to produce electronic equipment. One advantage to recycling metals is that they can be recycled repeatedly without any loss in quality. Despite its numerous benefits, urban mining faces financial, legal, and logistical challenges. First, the initial cost of constructing state-of-the-art recycling centers is extremely high. Thus, only a handful of smelters exist globally, which means e-waste must be transported far distances from around the world. Second, inconsistent legislation exists not only within the United States, but also around the globe. Because laws differ from region to region, enforcement becomes nearly impossible across borders. Third, for urban mining to be efficient, large quantities of e-waste are required. Low collection rates around the world mean many valuable materials are lost as a vast majority of e-waste is either thrown away with municipal waste by consumers or sent to developing countries for informal recycling. Fourth, companies lack the incentive to design products with end-of-life (EOL) in mind. Products are not designed to facilitate dismantling, to promote resource recovery, or to prevent eco- toxicity at their disposal. In fact, recent studies reveal an increasing trend of toxic metals in smartphones between 2007 and 2015 (Singh et al., 2019). To overcome these challenges coordination and cooperation must occur between the key players including individual governments, non-government organizations, informal and formal recyclers, manufacturers, and the public, who should take the following steps: enact and enforce federal legislation in the U.S., which includes ratifying The Basel Convention; coordinate laws and methods of compliance internationally, increase collection rates by offering more convenient and less expensive recycling options to consumers, incentivize companies to design less toxic products with EOL in mind, invest in research to improve all phases of e-waste recycling, and explore newer technologies such as non-toxic hydrometallurgical processes. TABLE OF CONTENTS EXECUTIVE SUMMARY………………………………………………………….….……….. i 1.0 INTRODUCTION……………………………………………………………………….. 1 1.1 What is E-waste?.............................................................................................................. 1 1.2 The Alarming Growth Rate of E-waste............................................................................ 3 1.3 Where is E-waste Generated?........................................................................................... 4 1.4 Where Does E-waste Go?................................................................................................. 5 1.4.1 China ............................................................................................................ 5 1.4.2 Other Developing Countries: India, Ghana, Nigeria .................................. 7 1.5 Negative Impacts of E-waste on the Environment ......................................................... 9 1.5.1 Soil .............................................................................................................. 10 1.5.2 Aquatic ecosystems ..................................................................................... 11 1.5.3 Air ............................................................................................................... 12 1.6 Negative Impacts of E-waste on Public Health ............................................................. 13 2.0 PHASES AND PROCESSES OF URBAN MINING 2.1 What is Urban Mining? ………………………………………………………………. 16 2.2 Collection …………………………………………………………………………….. 17 2.3 Pre-processing ……………………………………………………………………….. 18 2.3.1 Sorting and dismantling …………………………………………………. 18 2.3.2 Crushing, shredding, and milling ………………………………………... 18 2.3.3 Separation………………………………………………………………… 19 2.4 End-processing ……………………………………………………………………….. 19 2.4.1 Pyrometallurgical ……………………………………………………….. 20 2.4.2 Hydrometallurgical ……………………………………………………… 22 2.4.3 Biometallurgical …………………………………………………………. 23 3.0 BENEFITS OF URBAN MINING 3.1 Environmental ………………………………………………………………………… 25 3.1.1 Less harmful to environment than ore mining …………………………… 25 3.1.2 Preserves finite supply of rare earth elements …………………………... 26 3.1.3 Reduces amount of toxic waste released into environment ……………… 27 3.2 Human Health ………………………………………………………………………… 28 3.2.1
Details
-
File Typepdf
-
Upload Time-
-
Content LanguagesEnglish
-
Upload UserAnonymous/Not logged-in
-
File Pages67 Page
-
File Size-