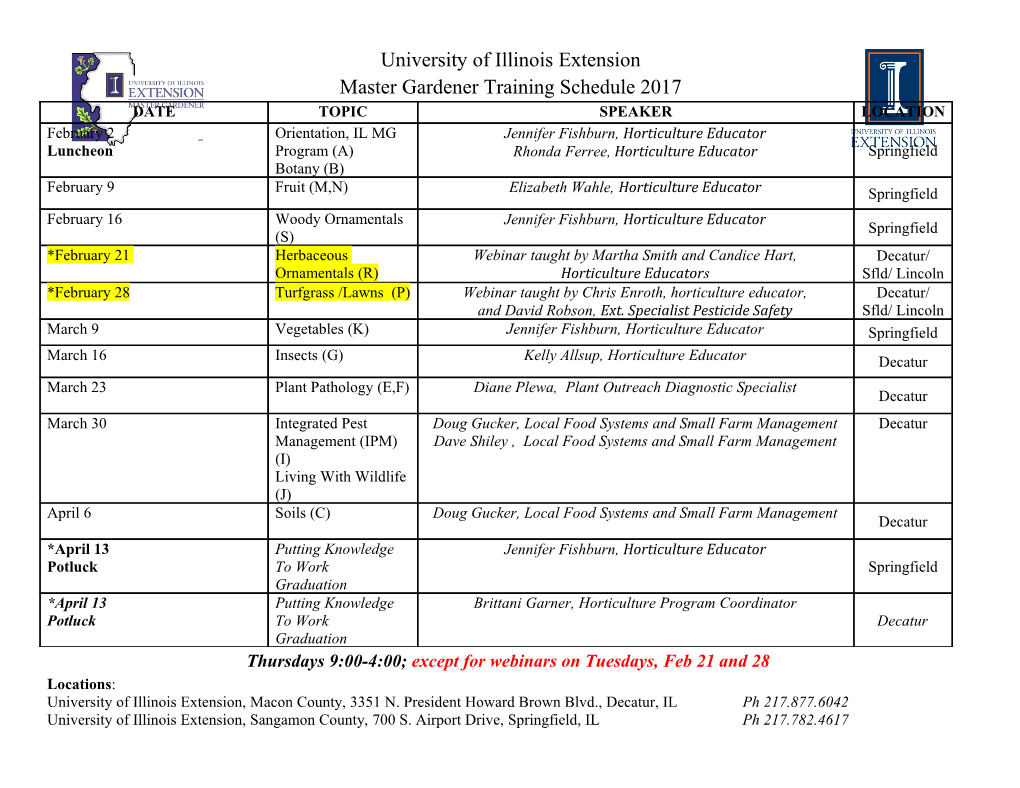
Allma Mater Studiiorum – Uniiversiità dii Bollogna DOTTORATO DI RICERCA IN Ingegneria Elettrotecnica Ciclo XXIX Settore Concorsuale di afferenza: 09/E2 Settore Scientifico disciplinare: ING-IND 33 PHYSICS OF ELECTRICAL TREEING IN SILICONE GEL Presentata da: Paolo Mancinelli Coordinatore Dottorato: Relatore: prof. Domenico Casadei prof. Andrea Cavallini Esame finale anno 2017 TABLE OF CONTENTS I THE THESIS WORK .................................................... 3 II SILICONE GEL............................................................ 5 II-1 POLYSILOXANES ............................................................................ 5 II-1.1 GENERAL CHARACTERISTICS ................................................... 5 II-1.2 APPLICATIONS ........................................................................ 9 II-1.3 SILICONE GEL PECULIARITIES ............................................... 10 II-2 POWER MODULES ENCAPSULATION ............................................. 11 II-3 CABLE JOINTS .............................................................................. 15 III PARTIAL DISCHARGE AND ELECTRICAL TREEING 18 III-1 PARTIAL DISCHARGES ................................................................. 18 III-1.1 PARTIAL DISCHARGE PHYSICS ............................................... 19 III-1.2 PARTIAL DISCHARGES DETECTION AND IDENTIFICATION ....... 21 III-2 ELECTRICAL TREEING IN SOLIDS ................................................. 26 III-2.1 TREE SHAPE .......................................................................... 26 III-2.2 TREE GROWTH STAGES AND PD ............................................. 29 III-2.3 ELECTRICAL TREE ON SILICONE GEL: PREVIOUS RESEARCHES ... ............................................................................................. 35 IV SAMPLE PRODUCTION AND EXPERIMENTAL SETUP 38 IV-1 SAMPLE MANUFACTURING ......................................................... 38 IV-2 EXPERIMENTAL SETUP ................................................................ 42 1 IV-2.1 MEASUREMENT CELL AND INSTRUMENTS .......................... 42 IV-2.2 TEST PROCEDURE................................................................ 44 V TREE INCEPTION TESTS.............................................. 47 V-1 INCEPTION IN SILICONE GEL ...................................................... 47 V-1.1 SINUSOIDAL AND SQUARE COMPARISON............................... 52 V-1.2 HIGH SLEW RATE .................................................................. 56 V-1.3 UNIPOLAR SQUARE VOLTAGE ............................................... 57 V-2 INCEPTION IN ELASTOMER .......................................................... 60 VI TREE INITIATION MODEL ....................................... 63 VI-1 SPACE CHARGE INJECTION ......................................................... 63 VI-2 CHARGE INJECTION MODEL ....................................................... 65 VI-3 CHARGE INJECTION CALCULATION ............................................ 68 VI-4 TREE INCEPTION MODEL ............................................................ 73 VI-5 MODEL VALIDATION ................................................................... 77 VII ELECTRICAL TREE GROWTH ................................. 82 VII-1 TIME AND LENGTH OF TREES ................................................. 82 VII-1.1 TIME TO BREAKDOWN (TTB) ................................................ 83 VII-1.2 MAXIMUM LENGTH AND FRACTAL NUMBER .......................... 86 VII-2 GROWTH MECHANISM IN GEL ................................................ 90 VIII CONCLUSIONS ......................................................... 96 REFERENCES ...................................................................... 99 2 I THE THESIS WORK The silicone gel use as electrical insulator is undoubtedly linked to the power electronic modules encapsulation. The combination of good thermal stability, electrical insulation, and mechanical characteristics is the reason of this link as it will be better explained in the next pages. Today, power modules encapsulation is still the major application of this material, even if other are growing of relevance, such as the insulation in cable joints. However, the progress of the technology in the power modules have been the triggering factor for several studies on the properties of this material. The increase of the operation voltage in power electronic modules brought a consequent increase of the electrical stress on the silicone gel used. In particular, the small size of the diodes and of the corners of the baseplate or the metallization causes very high field in particular areas. This is the classical condition for partial discharges and for a destructive degradation of the insulation such as the electrical treeing. The electrical trees have been deeply investigated in solid dielectric since the 70’s, but the behavior in the gel, that shows characteristics of both solid and liquid materials, is strongly different, as it will be explained in this work. This thesis divides the study on the electrical treeing into two main phases: the inception and the growth. Both are carried out assessing the influence of the waveform frequency and shape on the electrical treeing. The analysis of the tree inception, which is the initial stage of the tree, have a primary importance since it permits to evaluate the reasons and the conditions generating the tree. In this work, it is proposed a physical model explaining the mechanism of the inception, 3 supported by a numerical calculation and verified through the experimental tests carried out. The study of the electrical tree growth furnishes a knowledge of the rapidity and of the type of damage produced by the tree. In the particular case of the silicone gel, the evaluation of the growth permits to understand several peculiar features of this material, which make it highly different from solids. Although the electrical treeing is a well-known degradation in solids, it is not the same for gels. Therefore, this work wants to achieve the same level of understanding of the electrical treeing mechanism reached in solids also in gels. 4 II SILICONE GEL II-1 POLYSILOXANES The term “silicone” was chosen before the IUPAC rules by analogy with “ketone”. At that time, it was thought that the chemistry of silicone could have important analogies to the chemistry of carbon. The reason is, indeed, the similarity of the structural unit of the silicone base chain R2SiO with the ketone R2CO. According to IUPAC rules, the scientifically correct name for silicones is polyorganosiloxanes, from the siloxane chemical group Si-O-Si. Nevertheless, the term “silicones” is today commonly used for compounds in which silicon atoms are linked via oxygen atoms, where each silicon atom bearing one or several organic groups [1]. II-1.1 General characteristics Polysiloxanes present some peculiar features deriving from their general structure: 1. They are polymeric and they have the typical structural form of organic macromolecules. 2. They contain silicon-oxygen bonds, thus they have the same units of the structure forming silicates and silicic acids. 3. They contain hydrocarbon radicals linked directly to silicon and, therefore, they are correlated to organic chemistry. 5 For these features, silicones can be considered in an intermediate position between the inorganic and the organic compounds, with similitudes with both silicates and organic polymers. This dual nature causes the particular fascination of this class of compounds. A great variety of compounds is attainable in polyorganosiloxane chemistry. Indeed, different siloxane units can be combined with one another in the same molecule, leading to compounds with significant macroscopic differences. The various siloxane units can be divided according to the number of oxygen atoms bonded to the silicon one. Therefore, a single siloxane unit can be monofunctional (M), difunctional (D), trifunctional (T), or even tetrafunctional (Q) [2] as shown in Figure II-1. Figure II-1: Origin compound, functionality, and fields of application of silicone structural units from [2] Different composition of siloxane units may produce significantly different silicone products. Linear silicone fluids are composed mainly of D units, which give closed rings in combination with one another. The base polymers for silicone 6 elastomers or silicone rubbers consist of D units that bear cross-linkable functional groups. The main structural features of the highly branched silicone resins are T units, often combined with D units to make the resins more flexible. Silicone resins can also contain Q and M units. Actually, it is also possible to use combinations of three or four different types to construct a molecule, thus numerous silicone products can be synthesized and small ratio variations can significantly influence the final result. Despite the difference between the silicones, they have common properties: . A significant thermal stability and thermal resistance at high temperature, e.g. silicone elastomers have the widest operating temperature range of commercially important rubbers; . Low chemical reactivity with high oxidation resistance; . Anti-stick capability and good adhesion to surfaces with hydroxyl groups; . Low toxicity; . Low thermal conductivity, but it can be increased by a large amount of inert fillers; . The ability to repel water and form watertight seals; . Good resistance to oxygen, ozone, and UV light; . Gas Permeability; . Transparency; . Good electrical properties: Siloxanes
Details
-
File Typepdf
-
Upload Time-
-
Content LanguagesEnglish
-
Upload UserAnonymous/Not logged-in
-
File Pages112 Page
-
File Size-