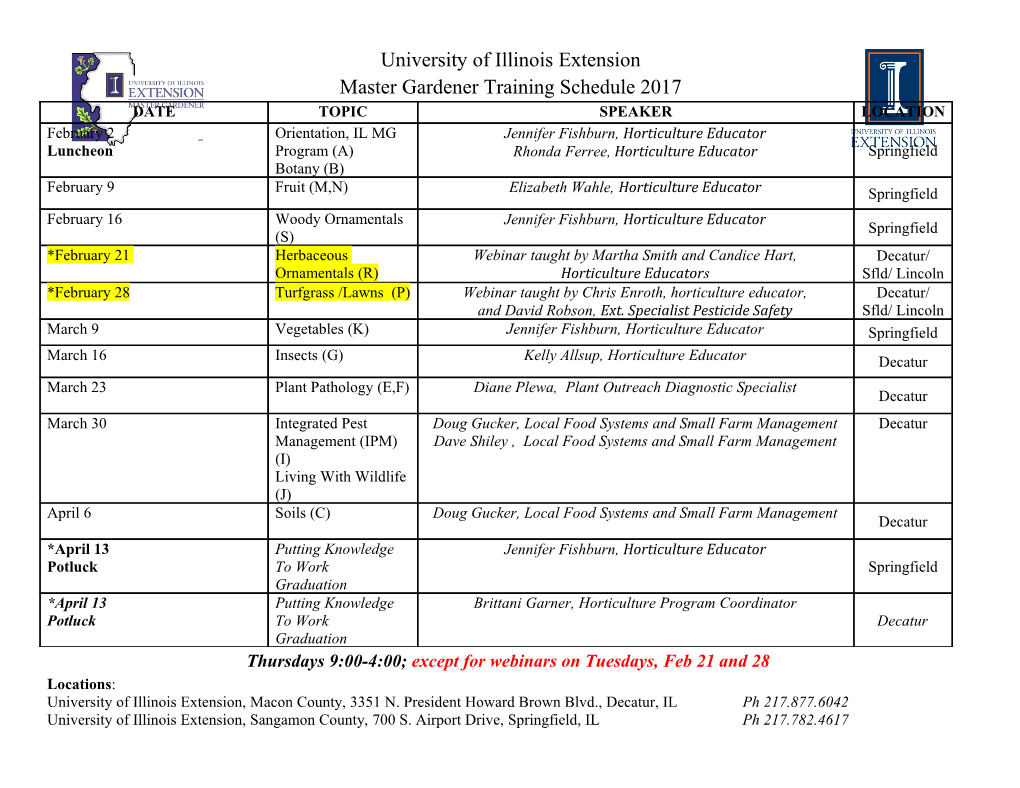
ISSUE 96 Dec 2009 Cooper Basin region now in 3D New 3D map assists geothermal exploration Tony Meixner The new 3D Cooper Basin map will aid explorers in a region By delineating the 3D geometries identified as highly prospective for geothermal energy. The newly of both the known high-heat produced map incorporates the two fundamental components which producing granites and inferred define a hot rock geothermal play: the potential heat source (high-heat granitic bodies that may be high producing granites) and the thermal insulation (overlying sediments). heat producing, and the overlying sedimentary basins, potential 140° 141° 142° hot rock geothermal plays are Canterbury identified. 0 100 km This study was carried QUEENSLAND out by Geoscience Australia’s Geothermal Project as part of 26° its Onshore Energy Security Program which provides pre- competitive information to support mineral and energy SOUTH AUSTRALIA resource exploration. 27° Durham Downs The Cooper Basin region The Cooper Basin region Innamincka straddles the Queensland/South 28° Australia border, and is coincident with a prominent anomaly on a map of predicted temperature at five kilometres depth (figure 1). The region forms part of a broad Data from Earth Energy Pty Ltd 09-4119-1 area of anomalously high heat Temperature at flow attributed to Proterozoic 5 km depth (°C) Drill hole location basement rocks enriched in NT 270 QLD naturally occurring radioactive WA SA elements. High-heat producing NSW granites, including granodiorite of VIC the Early to Mid-Carboniferous TAS 160 Big Lake Suite, intrude the Figure 1. Predicted temperature at five kilometres depth (Chopra & Holgate basement beneath the Cooper 2005) including well locations. and Eromanga basin sequences. Cooper Basin region now in 3D www.ga.gov.au/ausgeonews | 1 ISSUE 96 Dec 2009 These high-heat producing granites form a significant geothermal Cooper and Eromanga basins play being targeted by Australia’s first hot rock development by provide a thermal blanketing Geodynamics Limited at Habanero, near Innamincka in South effect resulting in temperatures Australia. as high as 270° C at depths of less than five kilometres. “The region forms part of a broad area of There is a high probability that corresponding geothermal plays anomalously high heat flow attributed to exist in association with other Proterozoic basement rocks enriched in granitic bodies lying beneath the Cooper and Eromanga basins. naturally occurring radioactive elements.” For the most part, the location and characteristics of these The relationship between high heat flow, high temperature bodies are poorly understood and gradient and anomalous heat production in the Big Lake Suite is accurately identifying them is an well recognised. The thick sedimentary sequences of the overlying important first step towards any future geothermal exploration in a) 140° 141° 142° this region. The Cooper region Bouguer gravity field is shown in figure 0 100 km 2a and the Z-horizon (top of QUEENSLAND basement) is shown in figure 2b. 26° 3 Two northeast-trending gravity e r u lows broadly coincide with the g i F Nappamerri and Tenappera troughs. These are bounded by SOUTH AUSTRALIA northeast-trending gravity highs which are similarly associated 27° with two structural ridges. In the northeast of the study gh rou a T arr ge area, two prominent structural haw Rid atc ncka gh P nnami rou - I rri T lows coincide with low gravity lia me me pa rri Nap Me anomalies. Thus, there is a broad - pa al regional correlation between 28° ge id e G Ridg ree known basin structure and the rte Mu e- ge h gravity field, suggesting that the on oug co Tr pa era ap app distribution of low-density basin N Ten sediments is of significance. There is, however, evidence 09-4119-2 that density variations in the Big Lake Suite Intersection basement are contributing to the Early Devonian Granite gravity field. Gravity lows that are -400 40 Drill hole location Bouguer Gravity (gu) coincident with the Nappamerri and Tenappera troughs extend Figure 2a. Gravity image showing drill-hole locations (Big Lake Suite beyond the trough boundaries intersections in white; early Devonian granite in red). The major structural elements of the Cooper Basin and the location of the north-south section in (figure 2b). A number of intense, figure 4 (white) are also shown. discrete gravity lows lie within Cooper Basin region now in 3D www.ga.gov.au/ausgeonews | 2 ISSUE 96 Dec 2009 b) 140° 141° 142° Constructing the 3D map 0 100 km The 3D map, which covers an QUEENSLAND area of 300 by 450 kilometres by 20 kilometres depth, was 26° constructed in part using 3D inversions of Bouguer gravity described by Li and Oldenburg SOUTH AUSTRALIA (1998). Gravity inverse modelling is a process whereby 27° adjustments are made to a density model, in the form of a mesh of gh ou Tr e rectangular prisms, until there rra Ridg wa cka ha amin ugh atc - Inn Tro is an acceptable fit between the P lia rri me ame rri app Me N predicted response of the model - a lp a e g and the observed gravity data. 28° id e G Ridg ree Where density observations rte Mu e - ge are available, it is possible to on h co ug pa Tro ap ra constrain the inversion to match N pe ap Ten the supplied density values to within a specified upper and 09-4119-3 lower bound. Where no density Big Lake Suite Intersection Drill hole location observations are available Early Devonian Granite the inversion can be left Figure 2b. Z-horizon image compiled from existing open file seismic unconstrained. sections, industry interpretations and 1300 well intersections (PIRSA 2008). The inversion model consisted This surface represents the base of the stacked Cooper and Eromanga basins. of a mesh with a cell size of two The Z-horizon ranges from 815 to 4496 metres below the topography. kilometres lateral and 250 metres the broader northeast trends, in some cases coinciding with granite vertical lengths. The smaller intersections in the Nappamerri and Tenappera troughs. In the vertical cell size used in this northwest of the study area where the Eromanga Basin lies directly on study was to accommodate the basement, there are a number of prominent gravity lows that are not high resolution of the Z-horizon associated with any known basin structure. (figure 2b) which represents the The gravity field is therefore influenced by both the thickness of base of the stacked Cooper and basin sediments and by density variations in the basement. For this Eromanga basins. Density values study, the gravity lows are interpreted as being sourced primarily from were assigned to the individual relatively low density granitic bodies that have intruded the basement. cells of the reference model based This assumption is supported by two lines of evidence. Firstly, all on whether the centre of the granite well intersections coincide with gravity lows and secondly, cell falls below the Z-horizon the nature of the basement which consists of Cambro-Ordovician (basement) or between the Warburton Basin and Early Palaeozoic and Proterozoic elements. Z-horizon and the topographic These basement units have been deformed and metamorphosed surface (Cooper and Eromanga to such an extent that the densities of the constituent units will be basin sediments). The inversions generally higher than granites that intrude these units. were forced to match the basin Cooper Basin region now in 3D www.ga.gov.au/ausgeonews | 3 ISSUE 96 Dec 2009 granitic bodies. If the density of the granite is too high and/or its depth extent is too low, then the inversion result will incorporate an anomalous region of low density in the basement directly beneath the modelled granite. Conversely, if the density of the modelled granite is too low Figure 3. North-south density section through the final gravity inversion and/or its depth extent is too model (see Figure 2a for location). The densities of the Eromanga Basin large, then an anomalous region sediments (dark blue: 2.3 +/- 0.2 g cm-3), Cooper Basin sediments (light blue: 2.5 +/- 0.2 g cm-3) and the granitic bodies (green: 2.6 +/- 0.2 g cm-3) of high density will be generated were constrained to a narrow density range, while the basement (yellow-red: at the base of the granite. The -3 2.65–2.75 g cm ) was left unconstrained. inversion model which produced the most ‘neutral’ result had a sediment density, 2.4 grams per cubic centimetre (g cm-3) based on a density of 2.6 g cm-3 assigned Bureau of Mineral Resources seismic refraction study, to within ± 0.2 to the modelled granites and g cm-3. Although a density was assigned to the basement (2.67 g cm-3), the corresponding upper and lower bounds were set such that they a maximum cut-off of 12 encompassed all likely rock densities. kilometres depth (figure 3). The inversion models of the basement have smooth variations in The final 3D map of inferred density. However, discrete boundaries can be constructed by producing sub-sediment granitic body 3D contour surfaces of the basement, termed iso-surfaces. A series distribution is shown in figure 4. of iso-surfaces were generated, based on a range of density values, enclosing successively larger regions of low density. The geometry of Conclusion the regions is lobe-like with the maximum lateral extent at or near The 3D map indicates that a the top of the basement and gradually reducing in lateral extent large volume of granitic material at depth. A gravity edge mapping technique was used to select the exists within the basement of the optimal iso-surface to constrain the sub-sediment lateral extent of Cooper and Eromanga basins.
Details
-
File Typepdf
-
Upload Time-
-
Content LanguagesEnglish
-
Upload UserAnonymous/Not logged-in
-
File Pages5 Page
-
File Size-