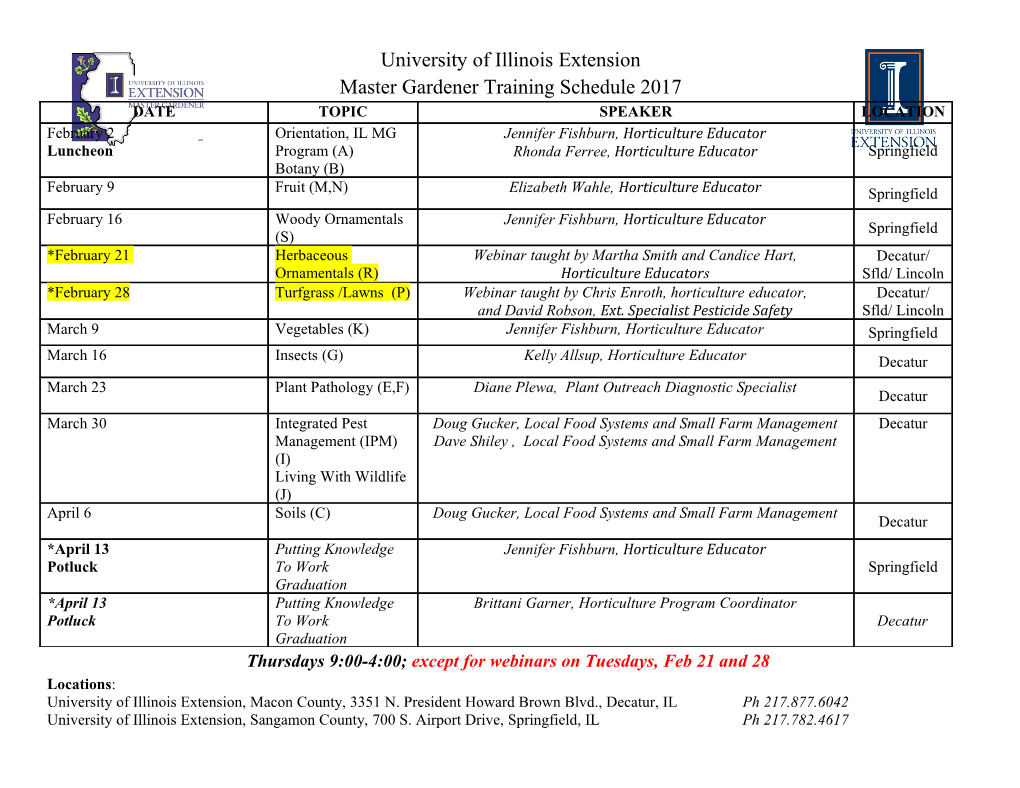
FINAL REPORT NASA GRANT NSG-2075 Covering the Period September i, 1975 To August 31, 1978 (NASA-CR-157784) (LUNAR MAGNETIC N79-10985 PEEMEABILITY, MAGNETIC FIELDS, AND ELECTRICAL CONDUCTIVITY TEMPERATURE] Final Report, I Sep. 1975 - 31 Aug. 1978 Unclas Jpanta Clra, Univ.)- 131 p_BC _A07/MF, A011 63/91 -35816Th . Submitted by Curtis W. Parkin Principal Investigator Department of Physics University of Santa Clara Santa Clara, California TABLE OF CONTENTS Page I. INTRODUCTION .... ......... 1i II. SCIENTIFIC RESULTS ............... ............ .... .. 2 A. Electrical Conductivity Temperature, and Structure of the Lunar Crust and Deep Interior .......... ............ 2 B. Lunar Magnetic Permeability and Iron Abundance; Limits on Size of a Highly Conducting.Lunar Core ...... ...... ..... 6 C. Lunar Remanent Magnetic Fields: Present-Day Properties; Interaction with the Solar wind; Thermoelectric Origin Model 8 III. PUBLICATIONS AND PRESENTED PAPERS . ........ ......... .... 12 APPENDIX - Publications Listed in Section III .......... 1 4 I. INTRODUCTION In the time period 1969-1972 a total of five magnetometers were deployed on the lunar surface during four Apollo missions. Data from these instru­ ments, along with simultaneous measurements from other experiments on the moon and in lunar orbit, have been used to study properties of the lunar interior and the lunar environment. The principal scientific results result­ ing from analyses of the magnetic field data are discussed in Section II. The results are presented in the following main categories: (1) Lunar elec­ trical conductivity, temperature, and structure; (2) Lunar magnetic permeabil­ ity, iron abundance, and core size limits; (3) the Local remanent magnetic fields, their interaction with the solar wind, and a thermoelectric genera­ tion model for their origin. In Section III relevant publications and presented papers are listed. Copies of publications resulting from the research funded by this grant are-included in the appendix. I. SCIENTIFIC RESULTS Magnetometers placed on the lunar surface and in orbit about the moon have returned a wealth of information about the moon which was not anticipated prior to the Apollo manned lunar missions. Analyses of lunar magnetic data have been used to study the following properties of the moon: Electrical conductivity, temperature, and structure of the lunar crust and deep interior; Lunar magnetic permeability and iron abundance, and inferred limits on size of a highly conducting lunar core; and-Lunar surface remanent magnetic fields: present-day properties, interaction with the solar wind, and origin by thermo­ electric generation. A. Electrical Conductivity "Temperature, and Structure of the Lunar Crust and Deep Interior Electrical conductivity of the lunar interior can be studied by analysis of two types of global induction fields: the poloidal field due to eddy currents driven by-time-varying external magnetic fields, and the toroidal -field due to unipolar currents driven through the moon by the motional solar. wind VxB electric field. Poloidal field induction has been used by many researchers to investigate lunar electrical conductivity, and to date poloidal induction analysis has yielded the most accurate conductivity informa­ tion at depths greater than 200 km. At shallower depths where the poloidal technique is limited by instrumental frequency response and number of measure­ ment sites, a toroidal induction analysis technique is used to determine the-­ upper limit of conductivity of the lunar crust. Since the conductivity is related to temperature, a global temperature profile can be calculated for an assumed compositional model of the lunar interior. Furthermore, structural properties of the-lunar interior can be inferred from characteristics of conductivity and temperature profiles and correlations with results from other geophysical instruments on the moon. 1. Conductivity at depths greater than 200 km. When the moon is in the solar wind, lunar eddy current fields form an induced lunar magnetosphere which is distorted in a complex manner due to flow of solar wind plasma past the moon. The eddy current field is compressed on the day side of the moon and is swept downstream and confined to the "cavity" on the lunar night side. Because of the complexity, early analysis -included a theory fortransient response of a sphere in a vacuum to model lunar response as measured on the lunar night side. An analysis-was also applied to time series magnetometer data taken on the lunar day side using a theory for transient response of a sphere immersed in a completely confining plasma. The most thorough and accurate analysis, using time-dependent poloidal response of a sphere in a vacuum, has been applied to data measured in the geomagnetic tail where plasma confinement effects are minimized. Two basic techniques have been applied to analysis of poloidal induction data measured with the moon ingtbe geomagnetic tail. The-first method used simultaneous measurements by the Apollo 12 lunar surface magnetometer (which measures total response field at the lunar surface) and an Explorer 35 orbiting magnetometer (which measures the externaldriving field). The second uses simultaneous measurements of- the total response field by-two surface magnetometers (Apollo 15 and 16 LSM's) and one close-orbiting-magnetometer (Apollo 16 subsatellite). The first-technique has been applied to the single largest transient measured with the moon in the geomagnetic tail; both techniques are applicable to aggregate "transient eventst9 made up of a linear superposition of -smaller events, Results of the different poloidal induction analysis techniques have been consistent, and an evolution-of improved computer analysis capabilities and use of more data as'it has become available, have yielded the following characteristics of the conductivity profile: The lunar conductivity rises rapidly with depth in the crust.to %,10- 3 nhhos/meter at a 200 to 300 km depth, which corresponds to the upper-mantle boundary reported in seismic results.- From 300 to 900 km depth the conductivity rises more gradually to 3xlO 2 mhos/m. 2. Conductivity of lunar crust; at depths less than 200 km. Studies of crustal conductivity have been completed using toroidal induction analysis. In the toroidal mode a unipolar current J is driven by an electric field -T E = VXBE which is produced as the solar magnetic field BE' frozen in the solar plasma, sweeps by the moon. V is the velocity of the moon relative to the solar plasma. Corresponding to the induced current JTis the toroidal field B which has a magnitude inversely proportional-to the total resistance to -=T current flow through.the moon; the magnitude of T (or likewise, AT) is limited by the region-of lowest conductivity in the current path, that is, the lunar crust0 An upper limit on the electrical conductivity of the lunar crust has been determined from upper limits on toroidal induction intthe moon by the solar wind VxB electric field. The toroidal induction theory is used for the spherically symmetric case of the induction field totally confined to the lunar interior or near-surface regions by a highly conducting plasma. Components of toroidal field are calculated by subtracting components of the external field BE measured by the Explorer 35-Ames magnetometer, from the total field B measured by the Apollo 12 lunar surface magnetometer By comparing the appropriate components of toroidal magnetic field and electric field, the upper limit of the proportionality factor relating these variables is determined. The qualityBTy,= BAy - BEy is compared to the electric field 5 component, Ez, which, is the largest of the three components for average solar wind conditions. These data are linearly correlated, with a least-squares slope A = C-6.2 ± 4.3) x 10- 2 sec/m, where the'limits include only random statistical measurement errors. Estimates of systematic instrumental errors are based upon comparisons between Apollo 12 LSM, Explorer 35-Ames, and r, Explorer 35-Goddard magnetometers. From this comparison we estimate the systematic error inherent in the analysis. W hen systematic ,errors due to uncertainties in instrumental gain factors are included, the upper limit slop becomes A - 2x10 - 7 sec/m. This factor is related to the average crustal conductivity upper limit of 0'crust"u10- 8 mhos/m for an assumed crustal thickness of 80 km. Wenote that the average crust conductivity is not a strong function of crust thickness for thicknesses 'U 80 km (e.g., a 100 km crust would correspond to a l.2x10 upper limit, and a 60 km crust would correspond to about 7x10- 7 mhos/m.) A very thin outer shell of even lower conductivity (indicated by radar and sample measurements for depths up to lb1 km) are consistent with this upper limit. The surface conductivity upper limit, derived from toroidal induction-analysis, places an important con­ straint on the previous lunar conductivity profile obtained from poloidal induction analysis: it lowers the previous crust conductivity limit nearly four orders of magnitude. Electrical conductivity profile results of the lunar interior are summarized in Figure 1. 3. Lunar temperature'and structure. The lunar temperature profile has been inferred from the electrical conductivity profile along with the experi­ mentally known dependence of the conductivity on the temperature for materials used to model the composition.of the lunar interior. For the thermal profile corresponding to the conductivity profile in Figure 1, assuming
Details
-
File Typepdf
-
Upload Time-
-
Content LanguagesEnglish
-
Upload UserAnonymous/Not logged-in
-
File Pages131 Page
-
File Size-