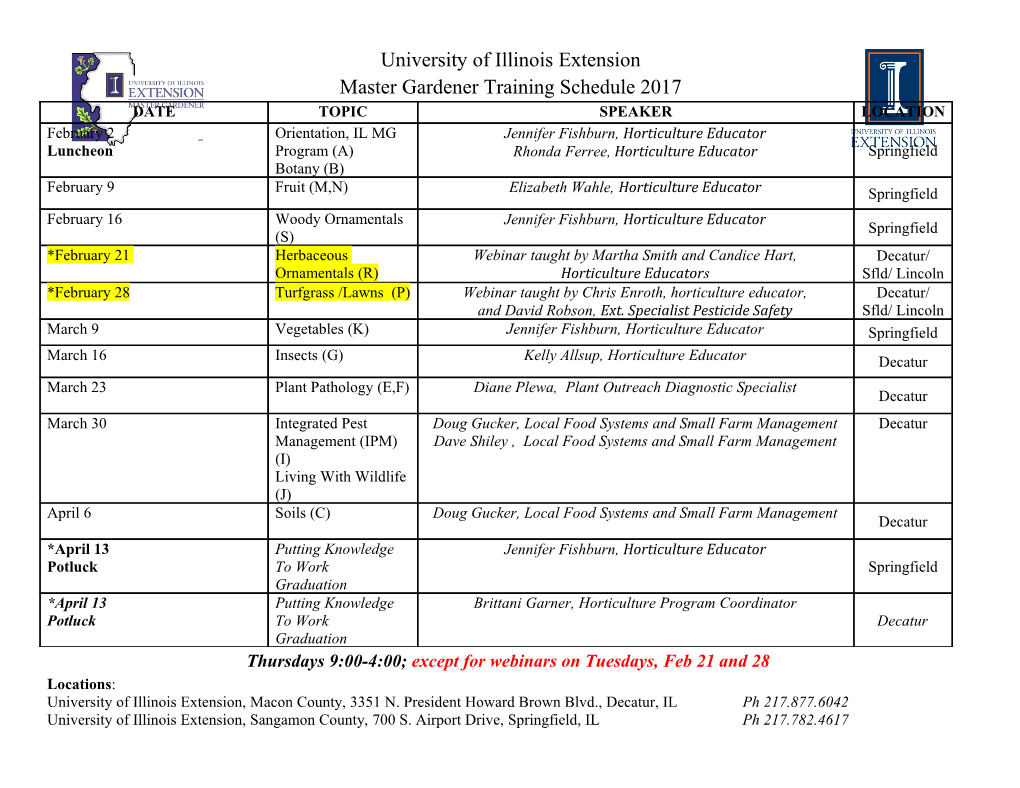
NUMERICAL STUDY OF THE HEAT TRANSFER IN A MINIATURE JOULE-THOMSON COOLER TEO HWEE YEAN NATIONAL UNIVERSITY OF SINGAPORE 2004 NUMERICAL STUDY OF THE HEAT TRANSFER IN A MINIATURE JOULE-THOMSON COOLER TEO HWEE YEAN (B.Tech Mech. Engrg (Hons.), NUS) A THESIS SUBMITTED FOR THE DEGREE OF MASTER OF ENGINEERING DEPARTMENT OF MECHANICAL ENGINEERING NATIONAL UNIVERSITY OF SINGAPORE 2004 ACKNOWLEDGEMENTS Acknowledgements There are many friends, colleagues and lecturers as well as institutions to whom I would like to express my thanks for their contribution and helpful information. I would like to express my thanks to Prof. Ng Kim Choon for his valuable comments and useful assistance regarding the topics in heat transfer of fluids and thermodynamics. I would also like to mention thanks for the kind foreword and the ideas and discussions from Assistant Prof. Chua Hui Tong and Dr Wang Xiaolin. Last but not least let me express my warmest thanks to the National University of Singapore and A*STAR for giving me the opportunity and full support, without which this project could not have been completed. Thank you. National University of Singapore i Filename:TeoHY.pdf TABLE OF CONTENTS Table of Contents PAGE Acknowledgements i Table of Contents ii-v Summary vi Nomenclature vii-x List of Figures xi-xiii List of Tables xiv Chapter 1 Introduction 1 1.1 Background 1 1.1.1 Recuperative Heat Exchanger 1 1.1.2 Regenerative Heat Exchanger 4 1.2 Present Trend 9 1.2.1 Open Cycle Cooling Systems 9 1.2.2 Inefficiencies & Parasitic Losses in Real Cryocooler 10 1.3 Objectives and Scopes 12 Chapter 2 Joule-Thomson Cooler Fundamentals 16 2.1 Parameters & Characteristics 19 2.1.1 The Flows 19 2.1.2 Capillary Tubes 23 2.1.3 J-T Coefficients & Throttle Valves 25 2.2 Refrigeration Cycle 29 National University of Singapore ii Filename:TeoHY.pdf TABLE OF CONTENTS 2.2.1 Stage 5 to 1 31 2.2.2 Stage 1 to 2 31 2.2.3 Stage 2 to 3 31 2.2.4 Stage 3 to 4 32 2.2.5 Stage 4 to 5 32 2.3 Hampson-Type J-T Cryostat 33 2.4 Experimental Model 37 Chapter 3 Governing Differential Equations 40 3.1 Geometry Model 40 3.1.1 Helical Coil Capillary Tube 40 3.1.2 Helical Coil Fins 41 3.2 High Pressure Cryogen in the Helical Coil Capillary Tube 48 3.3 Helical Coil Capillary Tube 50 3.4 Helical Coil Fins 50 3.5 Shield 51 3.6 External Return Cryogen 51 3.7 Spacers 53 3.8 Entropy Generation for Internal Cryogen 54 National University of Singapore iii Filename:TeoHY.pdf TABLE OF CONTENTS Chapter 4 Numerical Prediction 55 4.1 Computational Fluid Dynamics 55 4.2 Dimensionless Governing Differential Equations 57 4.2.1 High Pressure Cryogen (Single Phase Flow) 58 4.2.2 High Pressure Cryogen (Two Phase Homogenous Flow) 58 4.2.3 Helical Coil Capillary Tube 58 4.2.4 Helical Coil Fins 58 4.2.5 Shield 58 4.2.6 External Return Cryogen 59 4.2.7 Entropy Generation 59 4.3 Properties and Areas 60 4.3.1 Fanning Friction Factors 60 4.3.2 Convective Heat Transfer Coefficients 61 4.3.3 Thermodynamic and Transport Properties of Argon 61 4.3.4 Thermal Conductivities of Materials 68 4.3.5 Heat Transfer Areas 69 4.4 Boiling Heat Transfer 70 4.4.1 Nucleate Pool Boiling 71 4.4.2 Pool Film Boiling 74 4.4.3 Jet Impingement Boiling 75 Chapter 5 Results & Discussion 76 5.1 Temperature-Entropy (T-s) Diagram 77 National University of Singapore iv Filename:TeoHY.pdf TABLE OF CONTENTS 5.2 Cooling Capacity 79 5.3 Coefficient of Performance and Figure of Merit 83 5.4 Effectiveness and Liquefied Yield Fractions 84 5.5 Temperature and Pressure Distributions 86 Chapter 6 Conclusions & Recommendations 88 6.1 Conclusions 88 6.2 Recommendations 90 References R-1 Appendix A – Operation Manual for Simulation Program A-1 Appendix B – Fortran 90 Source Code – Main Program B-1 Appendix C – Fortran 90 Source Code – IMSL Subroutine (DBVPFD) C-1 Appendix D – Fortran 90 Source Code – IMSL Subroutine (FDJAC) D-1 National University of Singapore v Filename:TeoHY.pdf SUMMARY Summary The miniature Joule-Thomson (J-T) cooler is widely used in the electronic industry for the thermal management of power intensive electronic components because of special features of having a short cool-down time, simple configuration and having no moving parts. In this thesis, the sophisticated geometry of the Hampson-type J-T cooler is analyzed and incorporated into the simulation, so that the model can be used as a design tool. The governing equations of the cryogen, helical tube and fins, and shield are coupled and solved numerically under the steady state conditions, and yield agreements with the published experiments to within 3%. The characteristics of flow within the capillary tube and external return gas are accurately predicted. The temperature versus entropy, cooling capacity versus load temperature, and cooling capacity versus input pressure charts are plotted and discussed. The conventional way of simulating a Hampson-type J- T cooler, which is accompanied by a host of empirical correction factors, especially vis-à-vis the heat exchanger geometry could now be superseded. The effort and time spent in designing a Hampson-type J-T cryocooler could be greatly reduced. By avoiding the use of empirical geometric correction factors, the model produces the real behavior during simulation. National University of Singapore vi Filename:TeoHY.pdf NOMENCLATURE Nomenclature A Areas of contact m2 cp Isobaric specific heat J/(kg.K) Coef Heat Transfer Coefficient W/(m2.K) cv Isochoric specific heat J/(kg.K) D,d Diameter of tubes m ds Grid length along s-axis m d s Dimensionless grid length along s-axis - f Fanning friction factor - f(T,P) f is a function of T and P - G Mass velocity kg/(m2.s) h Specific enthalpy J/kg k Thermal conductivity W/(m.K) Ls Total length of capillary tube m m& Mass flow rate kg/s M Molecular Weight g/mol Mv Volumetric flow rate SLPM p Perimeter of heat transfer area m P Pressure Pa or N/m2 Pitchm Pitch of capillary tube m Pitchfin Pitch of fins m C µ Pr Prandtl number = p - k National University of Singapore vii Filename:TeoHY.pdf NOMENCLATURE q Heat transfer per unit mass W/kg Q& Heat transfer W Ro Universal gas constant J/(kg.K) ρUD Re Reynolds number = H - µ S& Specific entropy J/kg.s T Temperature K u Average velocity of fluid m/s x Quality of fluid - y Liqufied yield fraction - Greek Letters α Helical angle β Helical angle γ Non-linear coefficient λ Dimensionless conduction parameter µ Fluid dynamic viscosity µJ-T Joule-Thomson coefficient σ Stefan-Boltzmann constant ρ Fluid density ε Emissitivity θ Dimensionless temperature Φ Dimensionless pressure for hot fluid National University of Singapore viii Filename:TeoHY.pdf NOMENCLATURE ψ Dimensionless pressure for returned fluid Superscipts & Subscripts 0 Initial state 1,2,3,4,5 State points amb Ambient or room temperature and pressure conditions f High pressure incoming fluid fa High pressure vapor state in two-phase condition fl High pressure liquid state in two-phase condition finm Contact between capillary tube & fins (Area) fin Capillary fins fm Contact between high temperature fluid and capillary tube (Area) g Saturated fluid in gas state H Hydraulic hel Helical in Inlet l Low pressure returned fluid m Capillary tube man Mandrel min Minimum ml Contact between capillary tube and returned fluid (Area) out Outlet pc Polycarbonate National University of Singapore ix Filename:TeoHY.pdf NOMENCLATURE r Radiation ref Refrigeration s Shield sh Shield si Shield inside so Shield outside ss Stainless steel National University of Singapore x Filename:TeoHY.pdf LIST OF FIGURES List of Figures Page Figure 1.1 Classification of Recuperative Cycles Heat Exchangers 2 Figure 1.2 Regenerative Cycles Heat Exchanger 4 Figure 2.1 Joule-Thomson Cycle and Temperature-Entropy Diagram 19 Figure 2.2 Contours of velocity head non-dimensionalized with ½ρU2 21 Figure 2.3 Development of the axial velocity fields at Re=104 & Pr=7 21 Figure 2.4 Development of secondary velocity fields at Re=104 & Pr=7 22 Extracted from [21] Figure 2.5 Mean axial velocity distribution and vectors of means 22 secondary flows in curved and helically coil pipes Extracted from [20] Figure 2.6 The CFD models for the ordinary helix centerline 23 Extracted from [24] Figure 2.7 The streamline patterns near the top of the arch. 23 Extracted from [24] Figure 2.8 Typical J-T Cryostat Nozzle Schematic Diagram 26 Figure 2.9 Schematic of J-T Inversion Curve 28 Figure 2.10 Basic Joule-Thomson Cycle 30 Figure 2.11 A Real Hampson-type Joule-Thomson Cryocooler 35 Figure 2.12 Schematic of Hampson-Type Joule-Thomson Cryocooler 36 Figure 2.13 Schematic of Experimental Apparatus 37 Extracted from [12] National University of Singapore xi Filename:TeoHY.pdf LIST OF FIGURES Figure 2.14 Photograph of Experimental Apparatus Extracted from [12] 39 Figure 3.1 Helical Coil Notations – Capillary Tube 43 Figure 3.2 Helical Coil Notations – Fins 44 Figure 3.3 Elevation View of Helical Coil Capillary Tube and Fin 45 Figure 3.4 Plan View of Helical Coil Capillary Tube and Fin 46 Figure 3.5 Cross-Sectional View of Helical Coil Capillary Tube and Fin 47 Figure 3.6 Tf, Tl, Tm and Tfin Relations 50 Figure 4.1 Variation of Argon Density against Temperature 63 Figure 4.2 Variation of Argon Specific Heat against Temperature 63 Figure 4.3 Variation of Argon Entropy against Temperature 64 Figure 4.4 Variation of Argon Enthalpy against Temperature 64 Figure 4.5 Variation of Argon Viscosity against Temperature 65 Figure 4.6 Variation of Argon Thermal Conductivities against 65 Temperature Figure 4.7 Temperature-Entropy Charts for Argon 67 Figure 5.1 Simulated T-s Diagram (CASE 1) 78
Details
-
File Typepdf
-
Upload Time-
-
Content LanguagesEnglish
-
Upload UserAnonymous/Not logged-in
-
File Pages152 Page
-
File Size-