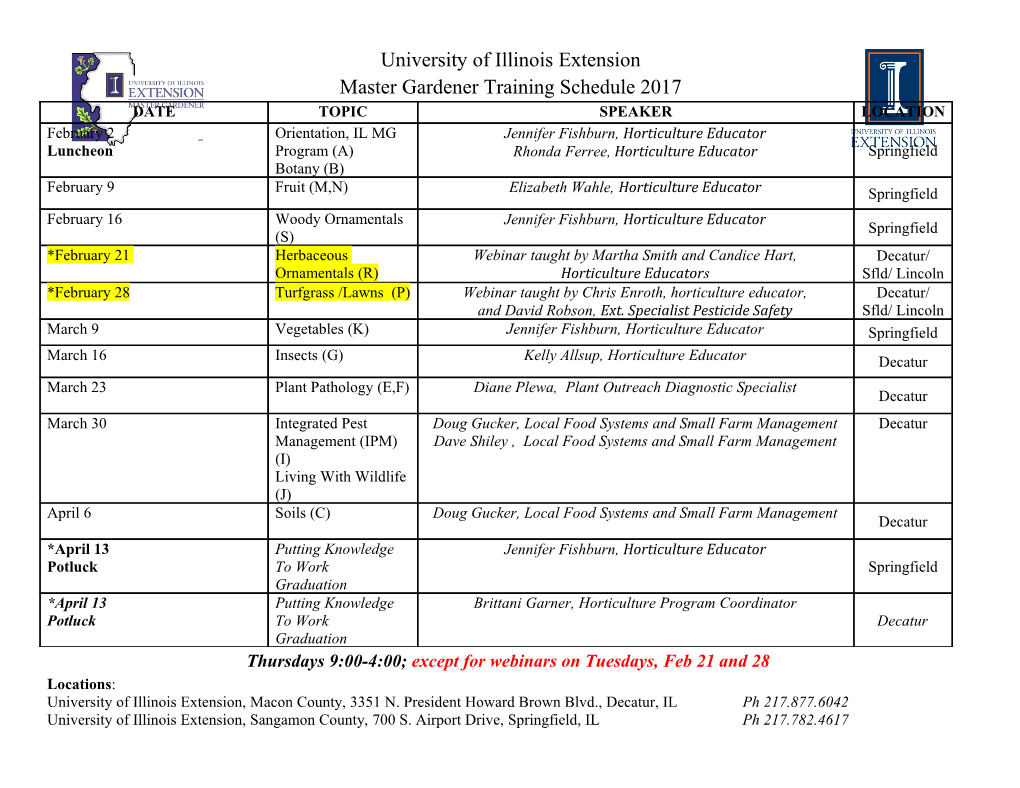
Appendix A Viewing the Real Projective Plane in R3;The Cross-Cap and the Steiner Roman Surface It turns out that there are several ways of viewing the real projective plane in R3 as a surface with self-intersection. Recall that, as a topological space, the projective plane, RP2, is the quotient of the 2-sphere, S 2,(inR3) by the equivalence relation that identifies antipodal points. In Hilbert and Cohn–Vossen [2](andalsodoCarmo [1]) an interesting map, H , from R3 to R4 is defined by .x; y; z/ 7! .xy; yz;xz;x2 y2/: This map has the remarkable property that when restricted to S 2,wehave H .x; y; z/ D H .x0;y0; z0/ iff .x0;y0; z0/ D .x; y; z/ or .x0;y0; z0/ D .x;y; z/. In other words, the inverse image of every point in H .S 2/ consists of two antipodal points. Thus, the map H induces an injective map from the projective plane onto H .S 2/, which is obviously continuous, and since the projective plane is compact, it is a homeomorphism. Therefore, the map H allows us to realize concretely the projective plane in R4 by choosing any parametrization of the sphere, S 2,and applying the map H to it. Actually, it turns out to be more convenient to use the map A defined such that .x; y; z/ 7! .2xy; 2yz;2xz;x2 y2/; because it yields nicer parametrizations. For example, using the stereographic representation of S 2 where 2u x.u; v/ D ; u2 C v2 C 1 2v y.u; v/ D ; u2 C v2 C 1 u2 C v2 1 z.u; v/ D ; u2 C v2 C 1 J. Gallier and D. Xu, A Guide to the Classification Theorem for Compact Surfaces, 105 Geometry and Computing 9, DOI 10.1007/978-3-642-34364-3, © Springer-Verlag Berlin Heidelberg 2013 106 A Viewing the Real Projective Plane in R3; The Cross-Cap and the Steiner Roman . Fig. A.1 Jakob Steiner, 1796–1863 we obtain the following four fractions parametrizing the projective plane in R4: 8uv x.u; v/ D ; .u2 C v2 C 1/2 4v.u2 C v2 1/ y.u; v/ D ; .u2 C v2 C 1/2 4u.u2 C v2 1/ z.u; v/ D ; .u2 C v2 C 1/2 4.u2 v2/ t.u; v/ D : .u2 C v2 C 1/2 Of course, we don’t know how to visualize this surface in R4, but we can visualize its four projections in R3, using parallel projections with respect to the four axes, which amounts to dropping one of the four coordinates. The resulting surfaces turn out to be very interesting. Only two distinct surfaces are obtained, both very well known to topologists. Indeed, the surface obtained by dropping y or z is known the cross-cap surface, and the surface obtained by dropping x or t is known as the Steiner roman surface. These surfaces can be easily displayed. We begin with the Steiner surface named after Jakob Steiner (Fig. A.1). 1. The Steiner roman surface. This is the surface obtained by dropping t. Going back to the map A and renaming x;y;z as ˛; ˇ; ,if x D 2˛ˇ; y D 2ˇ; z D 2˛; t D ˛2 ˇ2; it is easily seen that A Viewing the Real Projective Plane in R3; The Cross-Cap and the Steiner Roman . 107 xy D 2zˇ2; yz D 2x 2; xz D 2y˛2: If .˛;ˇ;/is on the sphere, we have ˛2 Cˇ2 C 2 D 1, and thus we get the following implicit equation for the Steiner roman surface: x2y2 C y2z2 C x2z2 D 2xyz: It is easily verified that the following parametrization works: 2v x.u; v/ D ; u2 C v2 C 1 2u y.u; v/ D ; u2 C v2 C 1 2uv z.u; v/ D : u2 C v2 C 1 Thus, amazingly, the Steiner roman surface (Fig. A.2) can be specified by fractions of quadratic polynomials! It can be shown that every quadric surface can be defined as a rational surface of degree 2, but other surfaces can also be defined, as shown by the Steiner roman surface. It can be shown that the Steiner roman surface is contained inside the tetrahedron defined by the planes x C y C z D 1; x y C z D 1; x C y z D 1; x y z D 1; with 1 Ä x;y;z Ä 1. The surface touches these four planes along ellipses, and at the middle of the six edges of the tetrahedron, it has sharp edges. Furthermore, the surface is self-intersecting along the axes, and has four closed chambers. A more extensive discussion can be found in Hilbert and Cohn–Vossen [2], in particular, its relationship to the heptahedron. One view of the surface consisting of six patches is shown in Fig. A.2. Patches 1 and 2 are colored blue, patches 3 and 4 are colored red, and patches 5 and 6 are colored green. A closer look reveals that the three colored patches are identical under appropriate rigid motions, and fit perfectly. Another revealing view is obtained by cutting off a top portion of the surface (Fig. A.3). 108 A Viewing the Real Projective Plane in R3; The Cross-Cap and the Steiner Roman . Fig. A.2 The Steiner roman 1 y surface 0.5 0 -0.5-1 1 0.5 0 z -0.5 -1 1 0 0.5 -1 -0.5 x Fig. A.3 A cut of the Steiner 1 roman surface 0.5 y 0 -0.5 -1 0.5 0 z -0.5 -1 -1 -0.5 0 x 0.5 1 In this picture, it is clear that the surface has chambers. We now consider the cross-cap surface. 2. The cross-cap surface. This is the surface obtained by dropping either the y coordinate, or the z coordinate. Let us first consider the surface obtained by dropping y. Its implicit A Viewing the Real Projective Plane in R3; The Cross-Cap and the Steiner Roman . 109 equation is obtained by eliminating ˛; ˇ; in the equations x D 2˛ˇ; z D 2˛; t D ˛2 ˇ2; and ˛2 C ˇ2 C 2 D 1. We leave as an exercise to show that we get .2x2 C z2/2 D 4.x2 C t.x2 C z2//.1 t/: If we now consider the surface obtained by dropping z, the implicit equation is obtained by eliminating ˛; ˇ; in the equations x D 2˛ˇ; y D 2ˇ; t D ˛2 ˇ2; and ˛2 C ˇ2 C 2 D 1. We leave as an exercise to show that we get .2x2 C y2/2 D 4.x2 t.x2 C y2//.1 C t/: Note that the second implicit equation is obtained from the first by substituting y for z and t for t. This shows that the two implicit equations define the same surface. An explicit parametrization of the surface is obtained by dropping z: 8uv x.u; v/ D ; .u2 C v2 C 1/2 4v.u2 C v2 1/ y.u; v/ D ; .u2 C v2 C 1/2 4.u2 v2/ z.u; v/ D ; .u2 C v2 C 1/2 One view of the surface obtained by cutting off part of its top part to have a better view of the self intersection, is shown Fig. A.4. 3: The Steiner roman surface, again. The last projection of the projective plane is obtained by dropping the x coordinate. Its implicit equation is obtained by eliminating ˛; ˇ; in the equations y D 2ˇ; z D 2˛; t D ˛2 ˇ2; and ˛2 C ˇ2 C 2 D 1. We leave as an exercise to show that we get 4.y2 C z2/t 2 D .z2 y2/.y2 z2 C 4t/: 110 A Viewing the Real Projective Plane in R3; The Cross-Cap and the Steiner Roman . Fig. A.4 A cut of the 1 cross-cap surface y 0.5 0 -0.5 -1 0.5 0 z -0.5 -1 -1 -0.5 0 x 0.5 1 This time, it is not so obvious that it corresponds to the Steiner roman surface. However, if we perform the rotation of the y; z plane by =4,wehave p p 2 2 y D Y Z; 2 2 p p 2 2 z D Y C Z; 2 2 and we have y2 C z2 D Y 2 C Z2 and z2 y2 D 2YZ. Thus, the implicit equation becomes 4.Y 2 C Z2/t 2 D 2YZ.2YZ C 4t/; which simplifies to Y 2t 2 C Z2t 2 C Y 2Z2 D 2YZt; which is exactly the equation of the Steiner roman surface. Just for fun, we also get the parametrization 4v.u2 C v2 1/ x.u; v/ D ; .u2 C v2 C 1/2 4u.u2 C v2 1/ y.u; v/ D ; .u2 C v2 C 1/2 4.u2 v2/ z.u; v/ D : .u2 C v2 C 1/2 A Viewing the Real Projective Plane in R3; The Cross-Cap and the Steiner Roman . 111 Fig. A.5 Another view of the 1 Steiner roman surface y 0.5 0 -0.5 -1 1 0.5 z 0 -0.5 -1 -1 -0.5 0 x 0.5 1 Fig. A.6 A cut of the Steiner 1 roman surface y 0.5 0 -0.5 -1 0.5 0 z -0.5 -1 -1 -0.5 0 x 0.5 1 This Steiner roman surface displayed in Fig.
Details
-
File Typepdf
-
Upload Time-
-
Content LanguagesEnglish
-
Upload UserAnonymous/Not logged-in
-
File Pages71 Page
-
File Size-