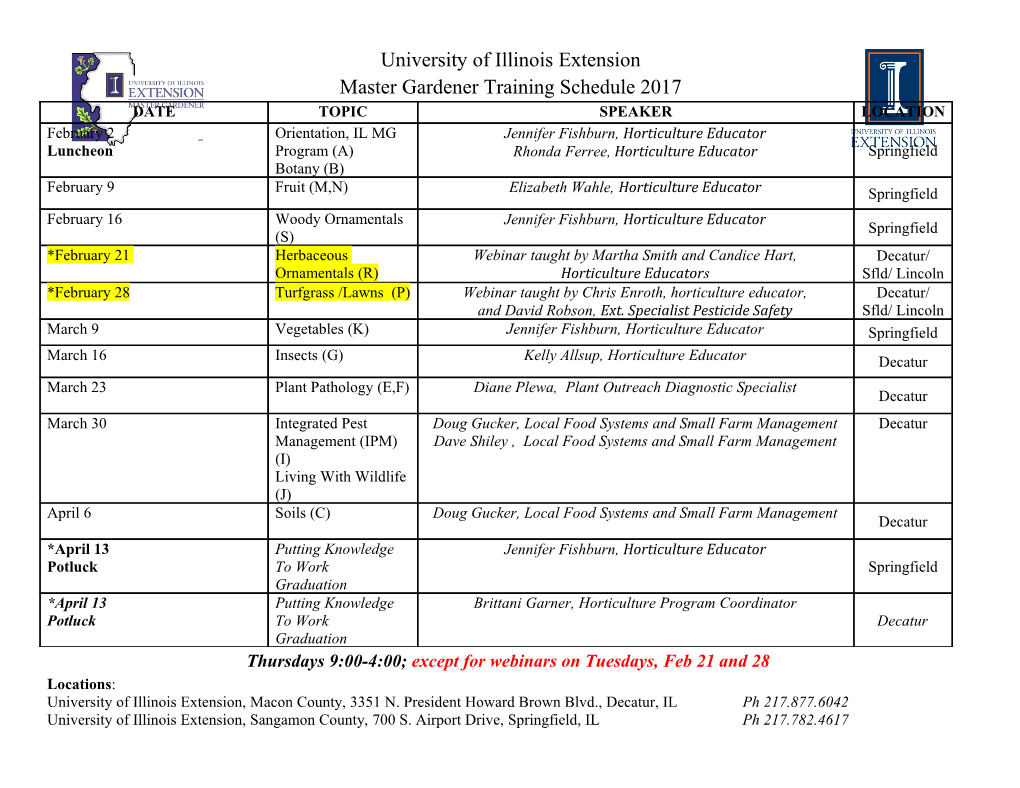
Entropy in molecular recognition by proteins José A. Caroa,1, Kyle W. Harpolea,1, Vignesh Kasinatha,1, Jackwee Lima,1, Jeffrey Granjaa, Kathleen G. Valentinea, Kim A. Sharpa, and A. Joshua Wanda,2 aJohnson Research Foundation and Department of Biochemistry and Biophysics, University of Pennsylvania Perelman School of Medicine, Philadelphia, PA 19104-6059 Edited by G. Marius Clore, National Institutes of Health, National Institute of Diabetes and Digestive and Kidney Diseases, Bethesda, MD, and approved May 9, 2017 (received for review December 26, 2016) Molecular recognition by proteins is fundamental to molecular free energy (ΔGtotal) of binding are measured and the total biology. Dissection of the thermodynamic energy terms governing binding entropy (ΔStotal) is determined by: protein–ligand interactions has proven difficult, with determina- tion of entropic contributions being particularly elusive. NMR re- ΔG = ΔH − TΔS = ΔH laxation measurements have suggested that changes in protein total total total total conformational entropy can be quantitatively obtained through − Δ protein + Δ ligand + Δ + Δ + Δ T Sconf Sconf Ssolvent Sr−t Sother . a dynamical proxy, but the generality of this relationship has [1] not been shown. Twenty-eight protein–ligand complexes are used to show a quantitative relationship between measures of fast side-chain motion and the underlying conformational entropy. Here and throughout the main text, the thermodynamic values We find that the contribution of conformational entropy can range refer to a standard 1 M state of concentration. The challenge is to from favorable to unfavorable, which demonstrates the potential quantify the various microscopic contributions to the free energy of of this thermodynamic variable to modulate protein–ligand inter- binding. Detailed atomic resolution structural models provide great actions. For about one-quarter of these complexes, the absence of insight into the origins of the enthalpy of binding. Much less certain conformational entropy would render the resulting affinity biolog- are the various contributions to the total binding entropy. In principle, several types of entropy are potentially important (right ically meaningless. The dynamical proxy for conformational entropy 1 or “entropy meter” also allows for refinement of the contributions side of Eq. ). Historically, entropy has most often entered the discussion in terms of the changes in the entropy of solvent water of solvent entropy and the loss in rotational-translational entropy Δ “ accompanying formation of high-affinity complexes. Furthermore, ( Ssolvent) and framed in terms of the so-called hydrophobic ef- fect” (10, 11). ΔSsolvent has, with some success, been related empir- structure-based application of the approach can also provide insight Δ into long-lived specific water–protein interactions that escape the ically to changes in accessible surface area ( ASA) of the protein and ligand upon complexation (12). Changes in the conformational generic treatments of solvent entropy based simply on changes in Δ Δ accessible surface area. These results provide a comprehensive and entropy ( Sconf) and the rotational-translation entropy ( Sr-t) (13) unified view of the general role of entropy in high-affinity molecu- of the interacting species have received far less attention, presum- lar recognition by proteins. ably because they have resisted experimental measurement. Con- tributions to binding entropy from unrecognized sources (ΔSother) 1 entropy | molecular recognition | binding thermodynamics | arealsoincludedinEq. and discussed below. protein dynamics | NMR relaxation Significance ost biological processes are controlled using molecular Mrecognition by proteins. Protein–ligand interactions regu- Molecular recognition by proteins is a key element of biology. late enzyme function, signaling pathways, and cellular events Appreciation of the underlying thermodynamics has been in- essential to life, particularly through allosteric mechanisms (1). complete because of uncertainty in several contributions to the Indeed, efforts at pharmaceutical intervention in disease have entropy. Here, we demonstrate a way to measure changes in largely centered on the manipulation of molecular recognition by protein conformational entropy using a dynamical proxy pro- vided by NMR relaxation methods. We find that conforma- proteins. The physical origin of high-affinity interactions in- tional entropy can contribute significantly and variably to the volving proteins has been the subject of intense investigation for thermodynamics of binding. In addition, we determine the con- decades. Structural analysis at atomic resolution has helped il- tribution of rotational-translational entropy loss upon forming a luminate in great detail the role played by enthalpy. On the other high-affinity complex involving a protein. The contribution of hand, the role of entropy in modulating the free energy of as- solvent entropy is also recalibrated. Thus, a more complete view sociation of a protein with a ligand has remained elusive. Of of entropy in binding has been established and shows that in- prime interest here is the conformational entropy of the protein, clusion of conformational entropy is necessary to understanding which is defined by the distribution of conformational states the origins of high-affinity interactions involving proteins. populated by the protein. A binding event can result in a re- distribution of populated states, and such differences are effec- Author contributions: A.J.W. designed research; J.A.C., K.W.H., V.K., J.L., K.G.V., and tively invisible in a static, monolithic view of proteins. It remains A.J.W. performed research; V.K., J.G., K.G.V., and A.J.W. contributed new reagents/ analytic tools; J.A.C., K.W.H., V.K., J.L., K.G.V., K.A.S., and A.J.W. analyzed data; and a challenge for structure-based efforts, agnostic to the confor- J.A.C., K.W.H., V.K., K.G.V., K.A.S., and A.J.W. wrote the paper. mational landscape available to the protein, to relate the inter- The authors declare no conflict of interest. actions of a few amino acids at a specific interface to the global, This article is a PNAS Direct Submission. experimentally measured free energies (2–4). The potential role Data deposition: The NMR chemical shifts and obtained order parameters have been for conformational entropy in protein function has been specu- deposited in the BioMagResBank, www.bmrb.wisc.edu (accession nos. 26667, 26670, lated about and simulated for some time (4–9), but a compre- 25728, 25727, 26983, 26619, and 26620). hensive, quantitative, and experimental evaluation of the extent 1J.A.C., K.W.H., V.K., and J.L. contributed equally to this work. and variation of its importance has been lacking. 2To whom correspondence should be addressed. Email: [email protected]. Experimental insight into binding entropy often begins from a This article contains supporting information online at www.pnas.org/lookup/suppl/doi:10. BIOPHYSICS AND calorimetric perspective where the heat or enthalpy (ΔHtotal) and 1073/pnas.1621154114/-/DCSupplemental. COMPUTATIONAL BIOLOGY www.pnas.org/cgi/doi/10.1073/pnas.1621154114 PNAS | June 20, 2017 | vol. 114 | no. 25 | 6563–6568 Downloaded by guest on October 2, 2021 Some time ago, it was realized that fast subnanosecond time constant]. There are now roughly two dozen published studies of scale motion between conformational states might provide ac- the change in methyl-bearing side-chain dynamics that are suf- cess to various thermodynamic features (14), especially confor- ficiently complete to use to test this idea (SI Appendix, Table S1). mational entropy (15, 16). Application of this idea has been Criteria for selection of these complexes included the availability thwarted by several technical limitations (17, 18), but has nev- of comprehensive assignments and relaxation data in both free ertheless led to the strong suggestion that dynamical proxies and complexed protein states, as well as a largely dry interface made available by NMR relaxation measurements could provide in the complex. We have augmented many of these NMR re- access to measures of conformational entropy (19). More re- laxation studies by measuring the binding thermodynamics using cently, efforts have been taken to overcome these technical isothermal titration calorimetry (ITC) under corresponding barriers and limitations and to render this approach to protein NMR solution conditions (i.e., buffer, pH, temperature). Com- entropy quantitative (20, 21). The resulting NMR-based dy- pletely new examples were also examined. The curated dataset is – namical proxy for conformational entropy or “entropy meter” summarized in SI Appendix, Tables S1 S4. takes a simple form that requires a few assumptions, particularly Previously, the empirically determined coefficients relating about the precise nature of the underlying motion (21): changes in accessible polar and apolar surface area to the sol- vation entropies of these two types of surface had to be derived h i Δ = proteinΔ 2 protein + ligandΔ 2 ligand subject to various assumptions about the nature of conforma- Stotal sd Nχ Oaxis Nχ Oaxis tional entropy (12). The unprecedented amount of dynamical data used here now allows us to determine the area coefficients + ΔSsolvent + ΔSr−t + ΔSother. directly. The data on the 28 proteins used here were obtained at [2] somewhat different temperatures (298–308 K). Significant heat 2 capacity changes accompany hydration of apolar and polar The first two terms contain
Details
-
File Typepdf
-
Upload Time-
-
Content LanguagesEnglish
-
Upload UserAnonymous/Not logged-in
-
File Pages6 Page
-
File Size-