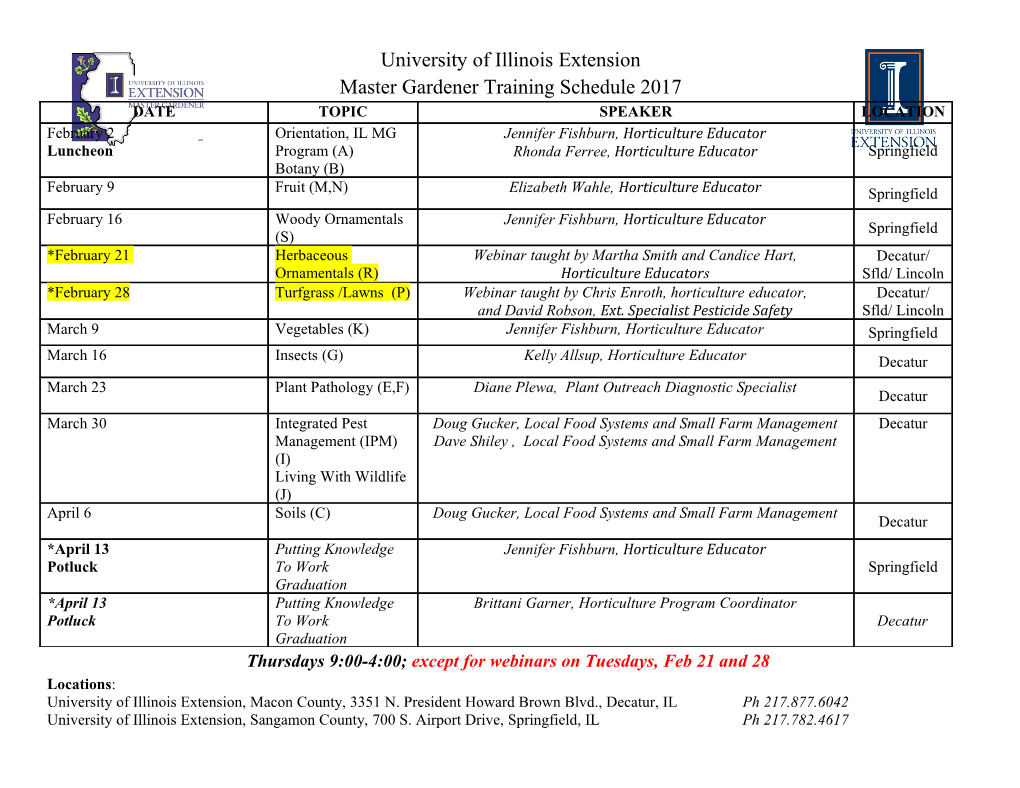
Modeling and Numerical Simulation of Partially Premixed Flames Ilona Zimmermann Universitat¨ der Bundeswehr Munchen¨ Fakultat¨ fur¨ Luft- und Raumfahrttechnik Thema der Dissertation: Modeling and Numerical Simulation of Partially Premixed Flames Verfasser: Ilona Zimmermann Promotionsausschuss: Vorsitzender: Prof. Dr. sc. techn. J¨orn Sesterhenn 1. Berichterstatter: Prof. Dr. rer. nat. Michael Pfitzner 2. Berichterstatter: Prof. Wolfgang Polifke, Ph.D. Tag der Pr¨ufung: 21.07.2009 Mit der Promotion erlangter akademischer Grad: Doktor der Ingenieurwissenschaften (Dr. Ing.) Neubiberg, den 16.11.2009 Acknowledgements This work was carried out at the University of Armed Forces Munich, Institute for Thermodynamics. I would like to express my sincere thank to Professor M. Pfitzner for his guidance and encouraging supervision during the entire project. He offered me the possibility to gain an insight to many different subjects. I am greatful to Professor Polifke who agreed to assist my work as co-examiner. I would also like to thank my colleagues of the Institute for Thermodynamics for interesting discussions, and my colleagues at ANSYS for the fantastic working atmo- sphere. Furthermore I would like to thank my friends who supported all the time. Danksagung Ein ganz besonderer Dank gilt nat¨urlich meiner Familie f¨urdie stetige Unterst¨utzung auf meinem Lebensweg und die Liebe die sie mir schenken. Vor allem mein Mann Mario gab mir stets den notwendigen R¨uckhalt und hat mich immer wieder auf meinem Weg best¨arkt. Abstract The demands on motor vehicles are steadily increasing. They have to be powerful and competitive but also environmentally friendly. This is not only due to the tight pol- lutant emission regulations, but also due to public interests. Although alternatives to internal combustion engines like hybrid or electro vehicles are under investigation and are already used for certain applications, it will take several decades until a wider use is possible. Thus conventional internal combustion engines have to be improved further. There is still potential like downsizing with supercharger, variable valve stroke or vari- able compression. But to be able to further improve the internal combustion engines, a deeper understanding of the combustion process is necessary. As experiments give only limited information, computational fluid dynamics (CFD) is a very powerful tool to gain more knowledge of the processes occurring within the engine and to improve the processes. In industry, the Eddy Dissipation Model is still a commonly used combustion model. As this is a very simple model, it is not successful to describe all the complex processes occurring in internal combustion engines. So this work focuses on the development of a suitable combustion model for direct injection engines in industrial applications. In such applications, combustion occurs under partially premixed conditions. The model presented is based on a flamelet concept with an additional transport equation for the reaction progress variable to account for the partially premixed regime. Different approaches are compared to close the source term of the reaction progress variable transport equation. The main effort has been spent for the correct description of the laminar flame speed, which changes with pressure, temperature and mixture composi- tion, which strongly vary in internal combustion engines. As the laminar flame speeds are derived in the context of premixed combustion, the validity range of the correlations is not wide enough for diffusion flames. So they are extended to the flammability limits, for which correlations are derived within this work to account for the pressure and temperature dependence. To also account for the tur- bulence effects, an effective laminar flame speed is introduced, used in pre-integrated tables, which also depends on the mean mixture fraction and mixture fraction fluctu- ation. The developed model is validated on a hydrogen jet flame, on a piloted methane- and heptane-air flame, and on a methane-air swirl burner. Especially for the hydrogen jet flame, and the piloted methane flame, a detailed set of experimentally obtained variables is available, like species mass fractions and temperature distributions. In comparison to the Eddy Dissipation Model, which is based on the assumption of a single step reaction, it could be seen that the Eddy Dissipation Model is not reliable enough to account for the complex processes occurring in internal combustion engines, whereas the flamelet model together with the reaction progress variable and the effective laminar flame speed shows promising and reliable results. Contents 1 Introduction 1 2 Fundamentals of Turbulent Reactive Flows 5 2.1 Characteristic Variables . 5 2.2 Flame Types . 7 2.3 Laminar Flame Speed . 13 2.4 Flammability Limits . 14 3 Basic Equations for Modeling of Non Reactive Flows 17 3.1 Instantaneous Equations . 17 3.2 Turbulent Flows . 19 3.3 Turbulence Models . 22 4 Modeling of Turbulent Reactive Flows 27 4.1 Eddy Dissipation Model . 31 4.2 The Laminar Flamelet Concept . 32 4.3 The Reaction Progress Variable . 38 4.4 Partially Premixed Flames . 41 5 H2 Jet Flame 44 5.1 Computational Domain and Boundary Conditions . 45 5.2 Influence of Chemistry Table . 47 5.3 Choice of Turbulence Model . 51 5.4 Implementation of Laminar Flame Speed . 52 5.5 Results and Discussion . 59 6 Sandia D Flame 69 6.1 Computational Domain and Boundary Conditions . 70 6.2 Influence of Chemistry Table . 71 6.3 Choice of Turbulence Model . 75 6.4 Implementation of Laminar Flame Speed . 75 6.5 Results and Discussion . 84 7 Low Swirl Burner 97 7.1 Computational Domain and Boundary Conditions . 98 7.2 Results and Discussion . 99 8 Conclusion and Outlook 110 8.1 Conclusion . 110 8.2 Outlook . 111 A The Presumed β-pdf Approach 113 B Flammability Limits 118 B.1 Hydrogen . 118 B.2 Methane . 120 C Combustion Chemistry 122 C.1 Methane Mechanism . 122 C.2 Hydrogen Mechanism . 131 D Additional Figures 132 D.1 H2 Jet Flame . 132 D.2 Sandia D Flame . 134 E Material Properties of Hydrogen 135 Nomenclature Latin Symbols A flame front surface area m2 c reaction progress variable – cp heat capacity at constant pressure J/(kg K) D diffusion coefficient m2/s G stretch factor – g gravity m/s2 H total enthalpy J h specific enthalpy J/kg k turbulent kinetic energy m2/s2 l length m m mass kg P probability density function p pressure P a R specific gas constant J/(kg K) s stoichiometric mass ratio – sl laminar flame speed m/s st turbulent flame speed m/s T temperature K t time s U diffusion velocity m/s u velocity m/s W molecular weight kg/mol X mole fraction – x space coordinate m Y mass fraction – Z mixture fraction – Greek Symbols χ scalar dissipation rate 1/s ε turbulent dissipation rate m2/s3 λ heat conductivity W/(Km) λ air excess ratio – µ dynamic viscosity kg/(ms) µt turbulent viscosity kg/(ms) ν kinematic viscosity m2/s ν0, ν00 stoichiometric coefficients; forward, backward reac- – tion ω source term or turbulent frequency φ equivalence ratio – ρ density kg/m3 τc chemical time scale s τf flow time scale s τfl flame time scale s τη Kolmogorov time scale s 2 τij stress tensor N/m Abbreviations CFD Computational Fluid Dynamics CPU Central Processing Unit DNS Direct Numerical Simulation JANAF Joint Army, Navy, and Air Force (USA) LES Large Eddy Simulation RANS Reynolds Averaged Navier Stokes Equation Dimensionless Numbers Da Damk¨ohlernumber Ka Karlovitz numer Le Lewis number Re Reynolds number Indices c chemical F fuel i species or space direction j species k species O2 oxidant q quenching st stoichiometric t turbulent u unburnt Chapter 1 Introduction Combustion is the oldest and most important energy conversion method worldwide. In 2006, the total world primary energy demand was 11,741 millions of metric tons of oil equivalent (Mtoe). Figure 1.1 shows the development of the world energy demand recorded by the International Energy Agency (IEA) [168]. Since start of recording in 1971 the energy demand has already doubled, and is still steadily increasing. 81 % of the energy conversion for domestic heating, power generation and transportation is due to the combustion of either oil, coal or gas. Energy sources like nuclear, hydro, solar or wind energy still play a minor role. Thus combustion of fossil fuels also remains a key technology in the foreseeable future. Besides the generation of heat for conversion to secondary energy like thermal, mechanical or electric energy, the combustion also produces pollutants like oxides of nitrogen (NOx) or sulfur, which are responsible for acid rain and effects on health. Pollutants like carbon dioxide are in the discussion to be responsible for global warming. Figure 1.1: World energy demand since 1971 [168]; **Other: geothermal, solar, wind, heat, etc Therefore, especially in the transportation sector, climate protection and saving resources has moved increasingly into public interests. Future automotive engines for example have to fulfill several requirements demanded by political conditions, social trends as well as the wish for competitive products in the global surroundings. Public discussions about climate change and lobbyists lead to increased public consciousness concerning costs and environment protection. Additionally the energy industry is de- pendent on the availability of fuel and thus is dependent on the few countries producing oil. In the foreseeable future the resources will decrease and the mineral oil output will reduce. The legislator also influences the public opinion and decisions as well as the requirements
Details
-
File Typepdf
-
Upload Time-
-
Content LanguagesEnglish
-
Upload UserAnonymous/Not logged-in
-
File Pages168 Page
-
File Size-