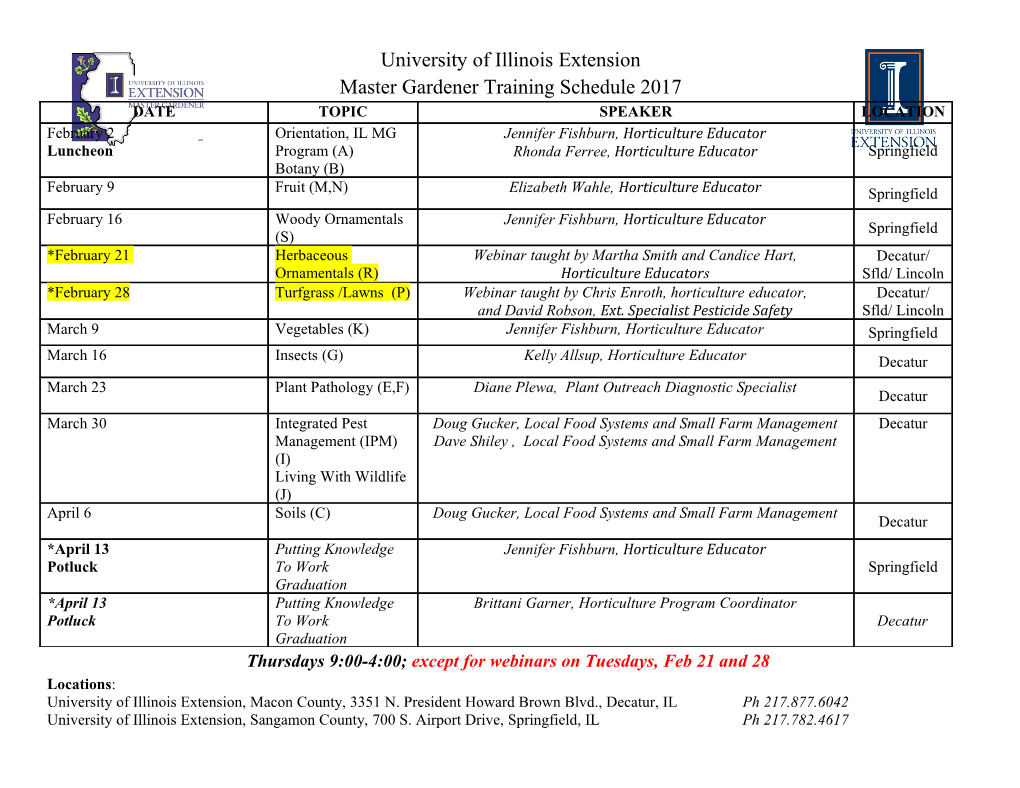
UNIVERSITY OF CINCINNATI Date: 30-Sep-2010 I, Brandon Michael De Graaf , hereby submit this original work as part of the requirements for the degree of: Master of Science in Nuclear & Radiological Engineering It is entitled: Determining the Effect of Shielding for an Eye Exposed to Secondary Particles Produced by Galactic Cosmic Rays using MCNPX Modeling Student Signature: Brandon Michael De Graaf This work and its defense approved by: Committee Chair: Henry Spitz, PhD Henry Spitz, PhD 10/29/2010 1,124 Determining the Effect of Shielding for an Eye Exposed to Secondary Particles Produced by Galactic Cosmic Rays using MCNPX Modeling A thesis submitted to the Graduate School of the University of Cincinnati in partial fulfillment of the requirements for the degree of Masters of Science in Nuclear Engineering of the College of Engineering and Applied Science by Brandon de Graaf B.S. Mechanical Engineering October 2010 Committee Chair: Henry Spitz, Ph. D. Committee Members: Samuel Glover, Ph. D; Bingjing Su, Ph. D; Thomas Huston, Ph. D. Abstract This thesis sets out to analyze the use of shielding to protect the lens of the eye from secondary particles produced by Galactic Cosmic Rays interacting with the structure of spacecrafts. The specific aim is to develop a model that will utilize a shield to reduce frontal dosage to an astronaut’s lens by 20%. In the MCNPX model, a proton beam will pass through an aluminum structure to create the secondary particles. Dosage to the lens will then be determined after the various shielding materials are placed between the eye and the source. iii This Page is Intentionally Left Blank iv Preface/Acknowledgement I want to thank the nuclear engineering staff at the University of Cincinnati, including Dr. Spitz, Dr. Su, and Dr. Glover, for their constant support and guidance throughout my undergraduate and graduate career. Without them this degree would not have been possible. I also wanted to thank Dr. Maldonado for introducing me to the field and inspiring my growth as a nuclear engineer. Lastly, I am dedicating this thesis in memory of Dr. Christenson. His passion for nuclear engineering resonated in all of his students and I am lucky to have enjoyed my time with him as my professor. v 1. Table of Contents Page Abstract iii Preface/Acknowledgement v List of Figures viii List of Tables ix Chapter 1. Introduction 1 Galactic Environment 1 Radiation Fundamentals 3 Radiation Protection 5 Effects on the Eye 6 2. Experiment 9 Methodology 9 MCNPX 11 Model Description 11 Shield Materials 14 3. Results 17 Secondary Particles Produced after Aluminum 17 Flux to the Eye Lens 17 Dose in the Eye Lens 18 Dose Reduction 19 4. Discussion 20 Breakdown of Results 20 Best Shield 21 Explanation for Higher Flux after Shield Materials 23 Benchmarking/ Realistic Results 23 Front Exposure vs. Back Exposure 24 Importance of the Results 25 Recommendations for Future Work 25 5. Conclusion 26 vi Bibliography 27 Appendices Appendix A: GCR Spectrum 29 Appendix B: Energy Spectrum of Secondary Particles after the Aluminum Wall 30 Appendix C: Front vs. Back Exposure 32 vii List of Figures Figure Page 1 The Three principal sources of space radiation 1 2 Production of secondary particles in aluminum 4 3 Dimensions (in millimeters) of the human eye 7 4 GCR spectra at the 1997 Solar Minimum and 1990 Solar Maximum 10 5 Diagram of geometry for MCNPX model 11 6 MCNPX model with eye blow up 12 7 Flux in the Eye Lens by secondary particle 17 8 Dose to the Eye Lens by secondary particle 18 9 MCNPX model of exposure from a beam through the back of the head 24 10 1997 GCR Spectrum used in MCNPX model 29 11a Energy Spectra by particle 30 11b Energy Spectra by particle 31 11 Front vs. Back Dose to the Eye Lens by secondary particle 32 viii List of Tables Table Page 1 Non-Shield Material Description 13 2 Shield Material Description 14 3 Thicknesses Required for 500 MeV Electron and 10000 MeV Proton – Selected Materials 15 4 Thicknesses Required for 500 MeV Electron and 10000 MeV Proton – Unselected Materials 16 5 Secondary Particles Produced after Aluminum Block 17 6 Lens Flux in particle/cm^2/proton After Each Shield Type 18 7 Lens Dose in MeV/g/proton After Each Shield Type 19 8 % Change in Total Dose as Compared to the No Shield Case 19 9 Dose in MeV/g/cm^2/month 22 10 Lens Dose in MeV/g/proton for Front and Back Exposure 32 ix 1. Introduction 1.1 Galactic Environment The galactic environment consists of two main sources: 1) Galactic Cosmic Rays (GCR) and 2) Solar Particle Events (SPE). Both sources include a dominant contribution of protons along with high energy (E) and high atomic number (Z) particles often referred to as HZEs (Nelson 2003). The separation between the two involves the energy spectrum and the origin of the sources; GCR originate from the galaxy, while SPE originate from our Sun. A third source is also found in Low Earth Orbit (LEO) and involves trapped electrons and protons in the Earth’s geomagnetic field, also known as Earth’s radiation belts. Fig 1: The three principal sources of space radiation: (1) galactic cosmic rays, (2) solar particle events, (3) trapped radiation in the Earth’s Radiation Belts. All three sources are affected by the Earth’s magnetic field. (E.R Benton, E.V. Benton 2001) Galactic Cosmic Rays can be thought of as the background radiation in space, as they are constantly being imposed on the spacecraft. The particles come from unknown sources in the galaxy, but its constituents are known and include 87% protons, 12% helium ion, and 1% heavier - 1 - ions. The intensity of GCR varies with the 11-year solar cycle. The solar cycle refers to the change in solar activity over the course of time. Solar winds attenuate GCR, meaning energy is removed through interactions. These solar winds, which are created from highly ionized gas emitted by the sun, are strongest during maximum solar activity. Thus GCR are weakest during solar maximums and strongest during solar minimums. Additionally, GCR are strongest near the Earth’s Poles. This is because GCR consists of charged particles and the magnetic attraction concentrates these particles near Earth’s poles. Current shielding techniques involve adding more equipment or structural material to the areas used most by astronauts. However, radiation shielding is usually considered at a later part in the spacecraft design, using less than optimum ways (Wilson et al. 2001). This means full protection from these particles is impractical due to large amount of material required, and thus large amount of weight. Therefore current research involves overhauling the design thought process and including lighter materials to protect astronauts. Solar Particle Events refer to particles released during rare but intense solar flares (E.R. Benton, E.V. Benton 2001). The particles released consist mostly of protons, with a small contribution from helium ions and heavier particles (NCRP 98 1994). Solar flares are infrequent and unpredictable, but occur during the active period of the solar cycle. Yet, not every solar flare produces particles of radiation concern, thus planning for radiation protection from this source is not an exact science. Current techniques involve moving astronauts to a more shielded area in the spacecraft when the SPE occur. These areas may be designed specifically with extra shielding, or involve rooms where there is enough equipment to act as a shield from the particles. - 2 - However, beyond these techniques it would be impractical to build a vessel with enough shielding for an event that might occur and only temporarily lasts. Trapped particles are charged particles that cannot escape the Earth’s magnetic field due to the intensity of the attraction. These trapped particles form a belt around the earth known as Earths radiation belt, also sometimes called the Van Allen belt. The belt consists of two distinct regions known as the inner and outer belts. The inner belt extends to about 2.8 times the Earth’s radius, while the outer extends from 2.8 to 12 times the Earth’s radii (NCRP 98 1994). These belts are not uniformly distributed and can change intensities with solar winds. In addition, intensity of the outer belt is much greater than the intensity of the inner. For radiation protection concerns, protons are the dominant charged particles. The major control to prevent doses is adjusting the vessel altitude. Additionally, mission plans will avoid high intensity areas in the belts and will alert astronauts if the vessel is about to temporarily enter a high intense region. For intergalactic travel GCR will account for the largest contribution of dose since it is a constant source for the astronauts. In addition, approximately half of the expected exposures on the International Space Station will be from GCR (Wilson et al. 2001). While SPE can cause more damage, they are infrequent and current shielding techniques will suffice. Therefore, this thesis will focus only on GCR and its respective secondary particles. Radiation Fundamentals Heavy charged particles, such as protons, lose energy by interacting with materials. The primary form of interaction occurs through the Coulomb force between the charged particles and the - 3 - atomic electrons or nuclei. This interaction removes tightly bound electrons from their orbits, causing the atoms to become charged or ionized. Another form of interaction is called a nuclear reaction, which occurs through the nuclear force with the nuclei. In this interaction, a charged particle will cause a neutron to be ejected from the nuclei. Generally, the probability of this reaction is low; however in the case of high-energy particles, such as those found in GCR, the reaction becomes more probable.
Details
-
File Typepdf
-
Upload Time-
-
Content LanguagesEnglish
-
Upload UserAnonymous/Not logged-in
-
File Pages41 Page
-
File Size-