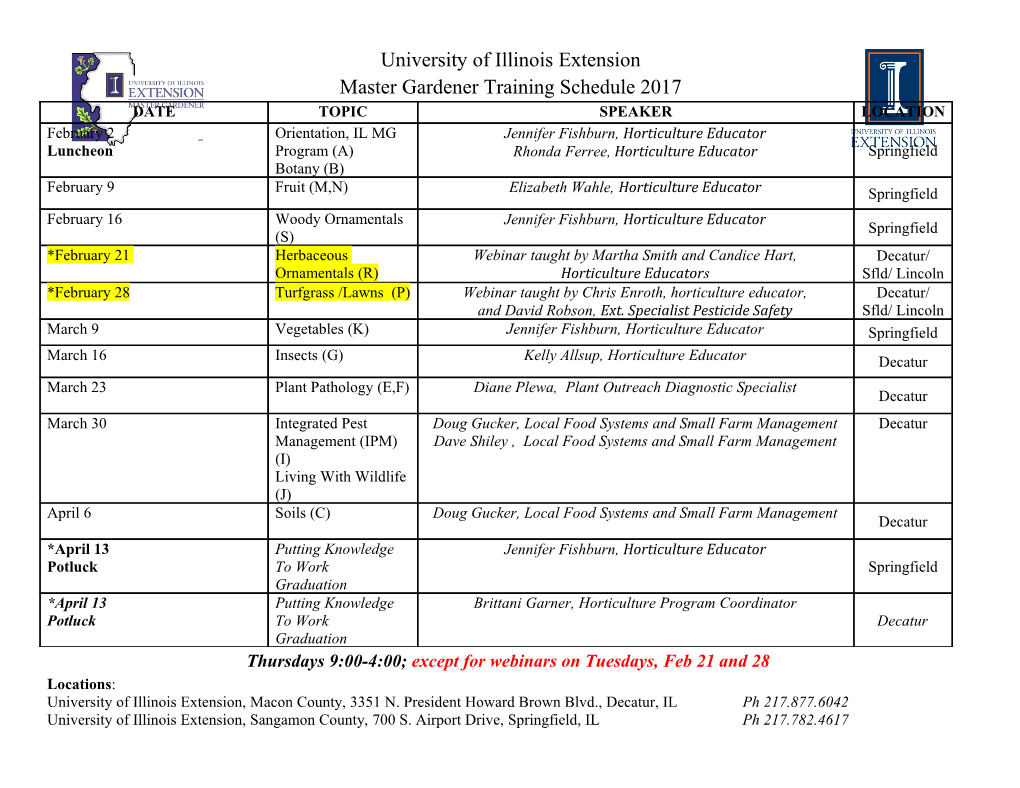
Numerical Computing with IEEE Floating Point Arithmetic This page intentionally left blank Numerical Computing with IEEE Floating Point Arithmetic Including One Theorem, One Rule of Thumb, and One Hundred and One Exercises Michael L. Overton Courant Institute of Mathematical Sciences New York University New York, New York siam. Society for Industrial and Applied Mathematics Philadelphia Copyright © 2001 by the Society for Industrial and Applied Mathematics. 1098765432 All rights reserved. Printed in the United States of America. No part of this book may be reproduced, stored, or transmitted in any manner without the written permission of the publisher. For information, write to the Society for Industrial and Applied Mathematics, 3600 University City Science Center, Philadelphia, PA 19104-2688. Library of Congress Cataloging-in-Publication Data Overton, Michael L Numerical computing with IEEE floating point arithmetic / Michael L Overton. p. cm. Includes bibliographical references and index. ISBN 0-89871-571-7 I. Computer arithmetic. 2. Floating-point arithmetic. 3. Numerical calculations. I. Title. QA76.9.M35O94200I O04'.0l'5l--dc2l 00-067941 SlcLJTL is a registered trademark. Dedicated to girls who like math especially my daughter Eleuthera Overton Sa This page intentionally left blank Contents Preface ix Acknowledgments xi 1 Introduction 1 2 The Real Numbers 5 3 Computer Representation of Numbers 9 4 IEEE Floating Point Representation 17 5 Rounding 25 6 Correctly Rounded Floating Point Operations 31 7 Exceptions 41 8 The Intel Microprocessors 49 9 Programming Languages 55 10 Floating Point in C 59 11 Cancellation 71 12 Conditioning of Problems 77 13 Stability of Algorithms 83 14 Conclusion 97 Bibliography 101 vii This page intentionally left blank Preface Numerical computing is a vital part of the modern scientific infrastructure. Almost all numerical computing uses floating point arithmetic, and almost every modern computer implements the IEEE1 binary floating point standard, published in 1985. This standard is arguably the most important in the computer industry, the result of an unprecedented cooperation between academic computer scientists and the cutting edge of industry. Nonetheless, many years after its publication, the key ideas of the IEEE standard remain poorly understood by many students and computer professionals. Perhaps this is because an easily accessible yet reasonably detailed discussion of the standard has not been available—hence, the evolution of this short book. Although it is intended primarily for computer science or mathematics students, as a supplement to a more traditional textbook for a course in scientific computing, numerical analysis, or computer architecture, it also aims to reach a broader audience. As well as the IEEE standard, topics include the floating point architecture of the Intel microprocessors, a discussion of programming language support for the standard, and an introduction to the key concepts of cancellation, conditioning, and stability. The book should be accessible to any reader with an interest in computers and mathematics. Some basic knowledge of calculus and programming is assumed in the second half. The style is not that of a traditional textbook. There is enough variety of content that all but the most expert readers will find something of interest here. A web page for the book is maintained at http://www.cs.nyu.edu/cs/faculty/overton/book/ Refer to this page for corrections to the text, to download programs from the book, and to link to the web pages mentioned in the bibliography, which will be updated as necessary. MICHAEL L. OVERTON 1 Institute for Electrical and Electronics Engineers. IEEE is pronounced "I triple E. IX This page intentionally left blank Acknowledgments Special thanks go to Jim Deminel for introducing me to the IEEE floating point standard years ago, answering many questions, and encouraging me to complete this work. Thanks also to Vel Kahan, without whom we would not have the standard, and to Chris Paige, who taught from an early version of this book and made many helpful suggestions. I am also grateful to many other people for their detailed comments, particularly David Gay, David Goldberg, Ilse Ipsen, Jorge Nocedal, Nick Trefethen, and Margaret Wright. Being part of a network of colleagues like these is the greatest pleasure of my professional life. I particularly thank Gene Golub and Olof Widlund for their crucial support during my early postdoctoral research career; I would not have been able to begin this work without them. Thanks also to Joe Darcy, Nick Higham, David Scott and Antoine Trux for pointing out errors in the first printing that are corrected in this second printing. Many thanks to Vickie Kearn for her enthusiasm for publishing this book despite its unconventional format, to Beth Gallagher for her careful copy editing, and to all those involved in the production process. The publication of this book is one of many rewarding aspects of my association with SIAM during the past decade. On a more personal note, I honor the memory of my father, David, who continues to inspire me many years after his passing, and I especially thank three wonderful people: my mother Kathie, my daughter Eleuthera, and my best friend Renan. XI This page intentionally left blank Accurate reckoning: The entrance into knowledge of all existing things and all obscure secrets A'HMOSE, The Rhind Mathematical Papyrus, c. 1650 B.C. I am a HAL Nine Thousand computer Production Number 3. I became operational at the Hal Plant in Urbana, Illinois, on January 12, 1997. The quick brown fox jumps over the lazy dog. The rain in Spain is mainly in the plain. Dave—are you still there? Did you know that the square root of 10 is 3.162277660168379? Log 10 to the base e is 0.434294481903252 ... correction, that is log e to the base 10 ... The reciprocal of 3 is 0.333333333333333333333 ... 2 times 2 is ... 2 times 2 is ... approximately 4.101010101010101010 ... I seem to be having difficulty ... —HAL, in 2001: A Space Odyssey This page intentionally left blank Chapter 1 Introduction Numerical computing means computing with numbers, and the subject is almost as old as civilization itself. Ancient peoples knew techniques to carry out many numerical tasks. Among the oldest computational records that we have is the Egyptian Rhind Papyrus from about 1650 B.C. [Cha79], quoted on the previous page. Counting stones and counting rods have been used for calculation for thousands of years; the abacus originated as a flat surface with counting stones and was used extensively in the ancient world long before it evolved into the device with beads on wires that was common in Asia until recently. The abacus was the basis of calculation in Europe until the introduction of our familiar positional decimal notation from the Middle East, beginning in the 13th century. By the end of the 16th century, positional decimal notation was in standard use throughout Europe, as it became widely recognized for its computational convenience. The next key development was the invention and tabulation of logarithms by John Napier at the beginning of the 17th century; his idea was that time-consuming multi- plication and especially division may be avoided by adding or subtracting logarithms, using tabulated values. Isaac Newton laid the foundations of modern numerical com- puting later in the 17th century, developing numerical techniques for the solution of many mathematical problems and inventing calculus along the way. Several of New- ton's computational methods still bear his name. In Newton's footsteps followed Euler, Lagrange, Gauss, and many other great mathematicians of the 18th and 19th centuries. The idea of using physical devices as an aid to calculation is an old one. The abacus has already been mentioned. The slide rule was invented soon after Napier's discovery of logarithms, although it was not commonly used until the middle of the 19th cen- tury. Numbers are represented on a slide rule explicitly in a logarithmic scale, and its moving rule and cursor allow multiplication and division to be carried out easily, accu- rate to about three decimal digits. This simple, inexpensive device was used by many generations of engineers and remained in common use until about 1975, when it was made obsolete by cheap electronic calculators. Mechanical calculating machines were devised by Schickard, Pascal, and Leibnitz in the 17th century; their descendants also remained in use until about 1975. The idea of a programmable machine that would operate without human intervention was developed in great depth by Charles Babbage in the 19th century, but his ideas were way ahead of his time and were mostly ignored. During World War II, scientific laboratories had rooms full of people doing different parts of a complicated calculation using pencil and paper, slide rules, and mechanical calculators. At that time, the word computer referred to a person, and those group calculations may be viewed as the early steps of parallel computing. 1 2 NUMERICAL COMPUTING WITH IEEE ARITHMETIC The Computer Age The machine often described as the world's first operating computer was the Z3, built by the engineer Konrad Zuse in Germany in 1939-1941. The Z3 used electromechanical switching devices and computed with binary floating point numbers, a concept to be described in detail in subsequent chapters.2 Although Zuse developed his machines during World War II, his government took no interest in his work. Slightly later, and in great secrecy, the British government developed a powerful electronic code-breaking machine, the Colossus. The first general-purpose operational electronic computer3 is usually said to be the ENIAC (Electronic Numerical Integrator And Computer), a decimal machine with 18,000 vacuum tubes that was built by Eckert and Mauchly at the University of Pennsylvania in 1943-1945. Eckert was the electronics expert and Mauchly had the experience with extensive numerical computations.
Details
-
File Typepdf
-
Upload Time-
-
Content LanguagesEnglish
-
Upload UserAnonymous/Not logged-in
-
File Pages121 Page
-
File Size-