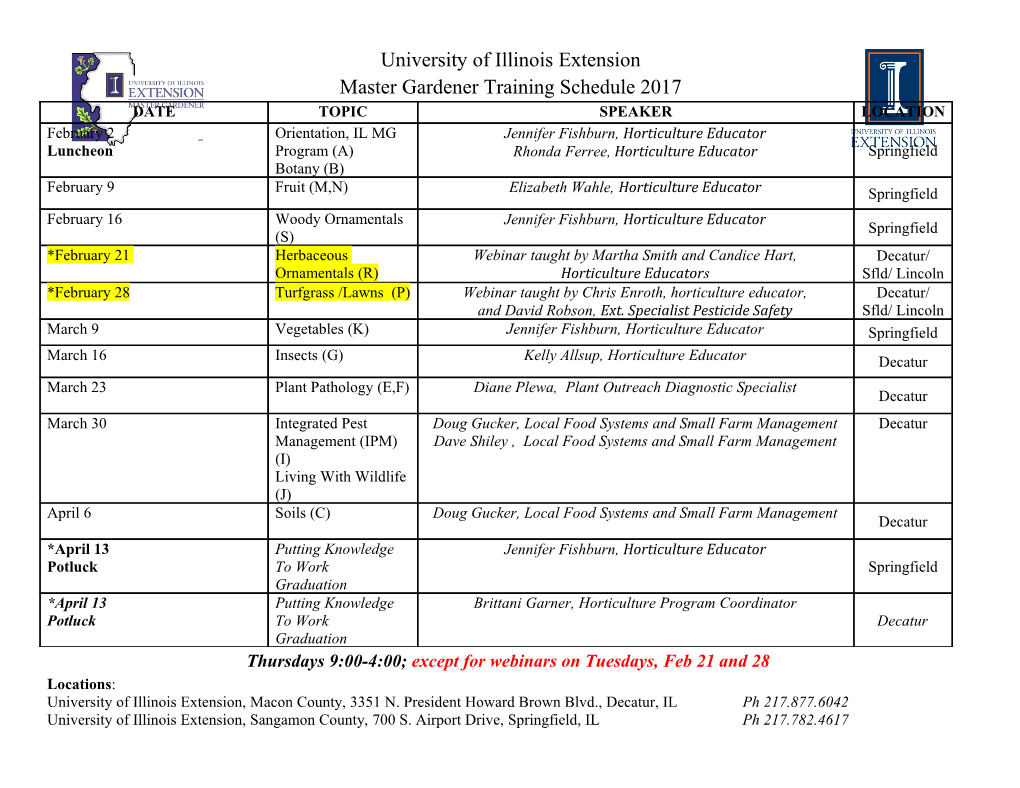
polymers Article Targeting Delivery System for Lactobacillus Plantarum Based on Functionalized Electrospun Nanofibers Hongliang Yu, Weihua Liu, Dongmei Li, Chunhong Liu , Zhibiao Feng * and Bin Jiang * Department of Applied Chemistry, Northeast Agricultural University, Harbin 150000, China; [email protected] (H.Y.); [email protected] (W.L.); [email protected] (D.L.); [email protected] (C.L.) * Correspondence: [email protected] (Z.F.); [email protected] (B.J.); Tel.: +86-451-5519-02-22 (Z.F.); +86-451-5519-09-74 (B.J.) Received: 30 June 2020; Accepted: 13 July 2020; Published: 15 July 2020 Abstract: With the increased interest in information on gut microbes, people are realizing the benefits of probiotics to health, and new technologies to improve the viability of probiotics are still explored. However, most probiotics have poor resistance to adverse environments. In order to improve the viability of lactic acid bacteria, polylactic acid (PLA) nanofibers were prepared by coaxial electrospinning. The electrospinning voltage was 16 kV, and the distance between spinneret and collector was 15 cm. The feed rates of the shell and core solutions were 1.0 and 0.25 mL/h, respectively. The lactic acid bacteria were encapsulated in the coaxial electrospun nanofibers with PLA and fructooligosaccharides (FOS) as the shell materials. Scanning electron microscopy, transmission electron microscopy, and laser scanning confocal microscopy showed that lactic acid bacteria were encapsulated in the coaxial electrospun nanofibers successfully. The water contact angle test indicated that coaxial electrospun nanofiber films had good hydrophobicity. An in vitro simulated digestion test exhibited that the survival rate of lactic acid bacteria encapsulated in coaxial electrospun nanofiber films was more than 72%. This study proved that the viability of probiotics can be improved through encapsulation within coaxial electrospun PLA nanofibers and provided a novel approach for encapsulating bioactive substances. Keywords: coaxial electrospinning; polylactic acid; probiotics; nanofibers 1. Introduction Probiotics are defined by the Food and Agriculture Organization of the United Nations and the World Health Organization (FAO/WHO) as living microorganisms that, when administered in adequate amounts, confer a health benefit on the “host” [1]. Probiotics have many functions, including the modulation of microbiota and metabolic activity in the gut, modulation of intestinal motility and absorption, impact on intestinal inflammation, modulation of the immune system and potential modulation of risk factors for cancer development [2]. Probiotics have been widely used in bioengineering, industry and agriculture, food safety, and life health [3,4]. However, most probiotics have poor resistance to adverse environments and are susceptible to temperature, oxygen, solvents, and mechanical oppression during processing. They are also susceptible to gastric acid and bile salts, which considerably reduce the amount of viable bacteria in the human digestive tract. These factors have ultimately made probiotics ineffective [5]. Microencapsulation has been found to be a useful tool for the stabilization of probiotic cells and bioactive substances in functional food applications. It can enhance the viability of probiotic cells during processing, storage, subsequent consumption, and Polymers 2020, 12, 1565; doi:10.3390/polym12071565 www.mdpi.com/journal/polymers Polymers 2020, 12, 1565 2 of 12 gastrointestinal digestion [6,7]. It has been reported that different methods such as emulsification, phase separation, and spray drying have been used to microencapsulate bioactive substances [7–10]. However, many of these methods involve high temperatures or a large amount of organic reagents, which may adversely affect the survival rate of probiotics or cause potential hazards to the human body. The electrospinning technique has been widely used to microencapsulate microorganisms and bioactive substances in recent years because it does not require severe temperature and pressure requirements [11–13]. Bioactive substances such as folic acid and fish oil have been encapsulated in electrospun fibers [14,15]. Microorganisms such as Escherichia coli, Staphylococcus epidermidis, and T4 bacteriophage have also been encapsulated in electrospun nanofibers [16,17]. Bifidobacterium has been encapsulated in protein (whey protein concentrate)/carbohydrate (pullulan) food hydrocolloids by means of the electrospinning technique [18] and Lactobacillus rhamnosus in poly (vinyl alcohol) and sodium alginate-based electrospun nanofibers [19]. Although electrospinning has been proved to be a promising process for encapsulating probiotics, little research has been done in this area. In previous studies, probiotics were encapsulated successfully and the survival rate of probiotics was significantly increased. However, most studies used blended electrospinning with a single base, and functional substances were rarely added to the embedding materials. In addition, many reports have confirmed that the addition of prebiotics can protect the activity of probiotics [20–22]. It can be reasonably suggested that adding prebiotics during electrospinning can further improve the survival ability of microencapsulated probiotics [23]. Polylactic acid (PLA) is usually synthesized through lactic acid fermentation. Lactic acid is a type of organic acid that is widely found in animals and plants; therefore, PLA has good biocompatibility [24]. PLA can be degraded in the human body, and the degradation product is directly metabolized by the human body to give the final products CO2 and H2O, which can be excreted out of the body through the kidneys. The US Food and Drug Administration (FDA) has long approved PLA use for absorbable surgical sutures, injection capsules, orthopedic fixation materials, microspheres for drug release, and tissue-engineered stents [25]. At present, PLA is considered as one of the most important materials with biodegradability and is widely used in the field of biomedicine [26,27]. In this study, the probiotics represented by lactic acid bacteria were encapsulated in PLA nanofibers by coaxial electrospinning, and fructooligosaccharides (FOS) were added as a prebiotic to improve the viability of probiotics in the digestive system. The physicochemical properties of the electrospun nanofibers were analyzed by means of scanning electron microscopy (SEM) micrographs, Fourier transform infrared spectroscopy (FT-IR), water contact angle, and differential scanning calorimetry (DSC). The survival rate of probiotics was studied via in vitro simulated digestion experiments. 2. Materials and Methods 2.1. Materials PLA (4032D) was purchased from NatureWorks LLC. (Minnetonka, MN, USA). Dichloromethane (DCM) and N,N-Dimethylformamide (DMF) were obtained from TianJin Fine Chemicals Co., Ltd. (TianJin, China). Disodium hydrogen phosphate (Na2HPO4) and potassium dihydrogen phosphate (KH2PO4) were purchased from Aladdin Reagent Co., Ltd. (Shanghai, China). Fructooligosaccharides (FOS), Rhodamine 123, and de Man Rogosa Sharpe (MRS) medium were obtained from Beijing − − Solarbio Science & Technology Co., Ltd. (Beijing, China). Lactic acid bacteria (Lactobacillus plantarum 1.0665) were donated by the College of Food Science, Northeast Agricultural University (Harbin, China). 2.2. Electrospinning Solution Preparation The shell solution was prepared by dissolving PLA in a mixture of DCM and DMF with a volume ratio of 7:3, and then FOS was added. The shell solution was magnetically stirred at room temperature (20–25 ◦C) for 6 h until it was dissolved completely. The concentration of PLA was 8% (w/v), and the concentration of FOS was 2.5% (w/v). The core solution was prepared by inoculating 1% (v/v) Polymers 2020, 12, 1565 3 of 12 L. plantarum 1.0665 into the MRS medium [23], which was cultured at 37 ◦C for 24 h. The concentration of the lactic acid bacteria was determined by the standard plate count and diluted to 108 CFU/mL. 2.3. Electrospinning Electrospinning was performed using a high-voltage direct power supply, and the shell and core solutions were consecutively filled in a 10-mL plastic syringe. A stainless steel needle with an inner diameter of 0.84 mm was used in shell electrospinning. A stainless steel needle with inner and outer diameters of 0.34 and 1.01 mm, respectively, was used in coaxial electrospinning. The feed rates of the shell and core solutions were 1.0 and 0.25 mL/h, which were controlled by syringe pumps. The electrospinning voltage was 16 kV, and the distance between spinneret and collector was 15 cm. The obtained electrospun nanofibers were collected using a grounded plate covered with a tin foil, and the resulting nanofiber films were stored in sterile boxes. The electrospinning process was carried out at 20–25 ◦C, and the relative humidity was around 40–60%. 2.4. Viscosity of Shell Solution The viscosity of shell solution was measured by a Brookfield digital viscometer (DV-S, AMETEK. Inc., San Diego, CA, USA) with an s61 rotor at 20 ◦C. The depth of the immersion solution was based on the groove above the probe [28]. The data were recorded when the number was stable. 2.5. Scanning Electron Microscopy (SEM) The electrospinning nanofiber films were cut out and adhered to the sample stage with a conductive tape. After sputtering Au and carbon coating with an ion sputtering apparatus, the microscopic morphology of the nanofibers were observed using a scanning electron microscope (SU 8010, Hitachi, Ltd., Tokyo,
Details
-
File Typepdf
-
Upload Time-
-
Content LanguagesEnglish
-
Upload UserAnonymous/Not logged-in
-
File Pages12 Page
-
File Size-