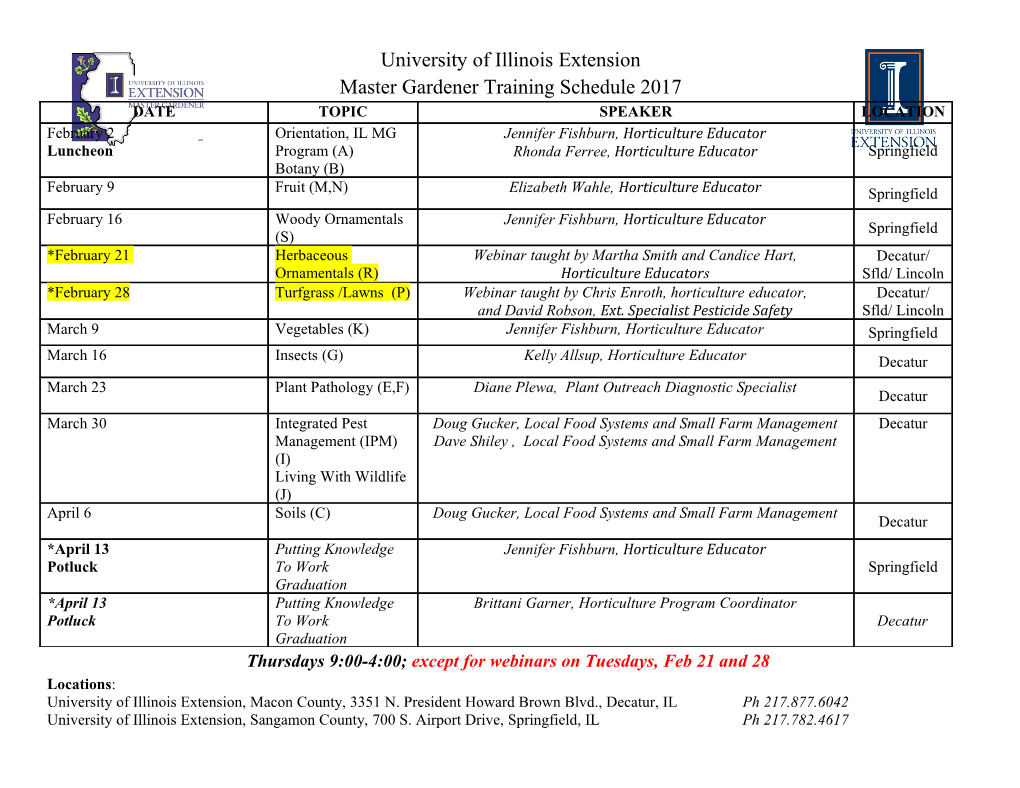
This file is part of the following reference: Bowden, Christopher David (2007) Epithermal systems of the Seongsan district, South Korea an investigation on the geological setting and spatial and temporal relationships between high and low sulfidation systems. PhD thesis, James Cook University. Access to this file is available from: http://eprints.jcu.edu.au/2040 Chapter 1 Chapter 1: Introduction to the epithermal systems of the Seongsan district, South Korea Bowden, C.D. Chapter 1: Introduction 1.1 INTRODUCTION The primary objective of this project is to investigate the geological setting and the spatial and temporal relationships between the distinctly different epithermal systems in the Seongsan district of South Korea. Detailed research aims are listed in Section 1.2 after some background information on selected issues for epithermal systems is presented and discussed. 1.1.1 Epithermal Au-Ag deposits and their related hydrothermal systems Epithermal Au-Ag deposits are an important source of precious metals, particularly within Phanerozoic orogenic belts of the circum-Pacific and southern Europe (Fig. 1-1). The large size and/or high grade of some epithermal Au-Ag deposits (Fig. 1-2) ensure that they continue to be an important exploration target. In addition to Au and Ag, epithermal deposits can also be an important source of base metals and clay-sulfate mineralisation (Kitagawa, 1999; Cooke & Simmons, 2000; Einaudi et al., 2003). Epithermal deposits are generally hosted in subaerial volcanic rocks within both island-arc and continental-arc settings (Cooke & Simmons, 2000; Sillitoe & Hedenquist, 2003; Simmons et al., 2005), and are generally inferred to have formed at relatively shallow levels in the crust (ca. <1.5km). They generally occur within calc-alkaline andesitic-dacitic-rhyolitic arcs or bimodal (basalt-rhyolite) volcanic suites, although systems hosted in alkalic rocks are also known (Richards, 1995; Jensen & Barton, 2000). They tend to form in low differential stress or extensional tectonic settings, although examples that formed in compressive and transpressive tectonic settings are also known (Sillitoe & Hedenquist, 2003). In addition, a variety of submarine-hosted epithermal systems have also been recognised more recently (Sillitoe et al., 1996; Sillitoe & Hedenquist, 2003; Stewart, 2003; Hannington et al., 2005). Epithermal deposits display a wide range of fluid chemistries, structural settings, alteration and ore mineral assemblages and mineralisation styles. This diversity of deposit characteristics is reflected in the numerous classification schemes that have been used in attempts to subdivide epithermal deposits (Table 1-1). The recent classification of epithermal systems, which is based on the sulfidation state of primary sulfides and results in subdivision into either high-sulfidation, intermediate-sulfidation or low-sulfidation types (Hedenquist et al., 2000; Table 1-1; Fig. 1-3), is perhaps losing popularity, with a trend back to descriptive nomenclature of individual deposits, based primarily on diagnostic hypogene gangue and alteration assemblages, but also describing ore minerals and deposit form (Cooke & Deyell, 2003; Simmons et al., 2005). Bowden, C.D. 1-2 Chapter 1: Introduction Seongsan Fig. 1-1 Distribution of principal Au-Ag epithermal deposits distinguished as either high- or low-sulfidation systems from: a), the circum-Pacific region; and b), Europe. From Hedenquist et al. (2000). The box around Korea represents approximate outline of Figure 1-8A. Bowden, C.D. 1-3 Chapter 1: Introduction Fig. 1-2 Grade-tonnage plot showing the size of epithermal Au mineralisation from deposits classified as either high- or low-sulfidation. From Hedenquist et al. (2000). High-Sulfidation systems: Bo=Boliden, Sweden; Ch=Chinkuashih, Taiwan; CP=Chelopech, Bulgaria; El=El Indio, Chile; El(DSO)=El Indio direct shipping ore, Chile, Go=Goldfield, Nev.; Le=Lepanto, Phil.; Mu=Mulatos, Mexico; Na=Nansatsu district, Japan; Pa=Pascua, Chile; Pi=Pierina, Peru; PP=Paradise Peak, Nev.; PV=Pueblo Viejo, DR; Ro=Rodalquilar, Spain; Su=Summitville, Col; Ta=Tambo, Chile; Ve=Veladero, Argentina; Ya=Yanacocha, Peru. Low-Sulfidation systems: Ba=Baguio, Phil.; CC=Cripple Creek, Nev.; CL=Comstock Lode, Nev.; Em=Emperor, Fiji; Hi=Hishikari, Japan; Ke=Kelian, Indo.; La=Ladolam, PNG; Mc=McLaughlin, Cal.; Mi=Midas, Nev; Po=Porgera, PNG; RM=Round Mountain, Nev.; SL=Sleeper, Nev.; Wa=Waihi, NZ. Bowden, C.D. 1-4 Chapter 1: Introduction Table 1-1 Classification schemes for epithermal systems. Modified from Sillitoe & Hedenquist (2003). classification scheme references descriptive nomenclature based on hypogene gangue and alteration minerals, ore metals, Cooke & Deyell (2003), deposit form and dominant Cu-bearing minerals Simmons et al. (2005) high-sulfidation intermediate-sulfidation low-sulfidation Hedenquist et al. (2000), Einaudi et al. (2003) Sillitoe & Hedenquist (2003) high-sulfidation high sulfide+base metal, low- low sulfide+base metal, low- Sillitoe (1989, 1993) sulfidation sulfidation type 1 adularia-sericite type 2 adularia-sericite Albino & Margolis (1991) alunite-kaolinite adularia-sericite Berger & Henley (1989) high-sulfidation low-sulfidation Hedenquist (1987) acid-sulfate adularia-sericite Hayba et al. (1985) Heald et al. (1987) high-sulfur low-sulfur Bonham (1986, 1988) hot-spring type Giles & Nelson (1982) enargite-gold Ashley (1982) epithermal Buchanan (1981) acid alkaline Sillitoe (1977) gold-alunite deposits gold-quartz veins in andesite: Lindgren (1933) argentite-gold quartz veins gold-quartz veins in rhyolite argentite veins gold telluride veins base metal veins gold selenide veins alunitic-kaolinic sericitic zinc-silver veins gold-silver-adularia veins, Emmons (1918) veins fluoritic tellurium-adularia gold veins goldfield type Ransome (1907) Bowden, C.D. 1-5 Chapter 1: Introduction HS=high-sulfidation; IS=intermediate-sulfidation; LS=low-sulfidation. Fig. 1-3 Proposed schematic sections of end-member volcanotectonic settings and commonly associated epithermal and related mineralisation types from Sillitoe & Hedenquist (2003). In general, high-, intermediate- and low- sulfidation epithermal systems do not occur in close proximity to each other although each is part of a broad spectrum of epithermal-type systems found in volcanic-hypabyssal settings. For an explanation of hydrothermal plumbing in epithermal systems, refer to Figure 1-6. a. Stratovolcano within a calc-alkaline volcanic arc under a low differential stress to mildly extensional tectonic setting showing relationships between high- and intermediate-sulfidation epithermal (and porphyry) systems. This style of mineralisation would also be applicable within a dome complex. b. Rift setting with bimodal volcanism and low-sulfidation deposits. Note: low-sulfidation deposits can also occur within extensional arcs characterised by andesitic-dacitic-rhyolitic volcanism (i.e. setting a.). In addition, submarine examples of the above settings are now proving to show significant epithermal potential (Sillitoe et al., 1996; Sillitoe & Hedenquist, 2003; Stewart, 2003). Bowden, C.D. 1-6 Chapter 1: Introduction Descriptive methods address some of the limitations encountered with using sulfidation-state classification. The dominant fluid or mineral-assemblage sulfidation-state has generally been used to classify a particular deposit as either high-, intermediate- or low-sulfidation epithermal deposit. However many epithermal systems can show localised to broad-scale variations in mineral sulfidation state due to factors such as either boiling or local wall-rock influence (Einaudi et al., 2003). This classification typically does not reflect the fluid complexities, localised variations in sulfidation state or overprinting characteristics within a given system. Additionally, systems with low sulfide content are not easily classified using this system, although alteration assemblages and/or vein textures are typically used to correlate with other systems (Table 1-2). Gangue and alteration minerals are generally present in a hydrothermal system and their mineralogy can be used to infer conditions of formation such as temperature, pressure and fluid composition. Alteration and gangue minerals can be analysed across the entire epithermal system, from peripheral alteration to core mineralisation zones throughout the paragenesis, whereas the sulfidation state of ore sulfides (if present!) only indicate the ore-fluid sulfidation state. Therefore, initial examination of epithermal systems is probably best undertaken by documenting the gangue and alteration mineral assemblages and their paragenesis (White & Hedenquist, 1995; Cooke & Deyell, 2003; Simmons et al., 2005). This will indicate the conditions of formation for the system. Then if ore sulfides are present, these can be examined to constrain the sulfidation-state of the ore-forming fluids (Hedenquist et al., 2000). 1.1.1.1 Comparison of sulfidation-state with descriptive models for epithermal systems Ore mineral assemblages in high-sulfidation systems, by definition, include sulfides of high sulfidation state, such as enargite, luzonite, covellite-digenite, famatinite and orpiment (Table 1- 2). Alteration assemblages that commonly form in association with the development of high- sulfidation mineralisation include alunite, quartz, pyrophyllite, kaolinite and rarely diaspore, andalusite, and zunyite. These high-sulfidation systems are analogous to acid-sulfate systems (Table 1-1). High-sulfidation systems form from oxidised,
Details
-
File Typepdf
-
Upload Time-
-
Content LanguagesEnglish
-
Upload UserAnonymous/Not logged-in
-
File Pages39 Page
-
File Size-