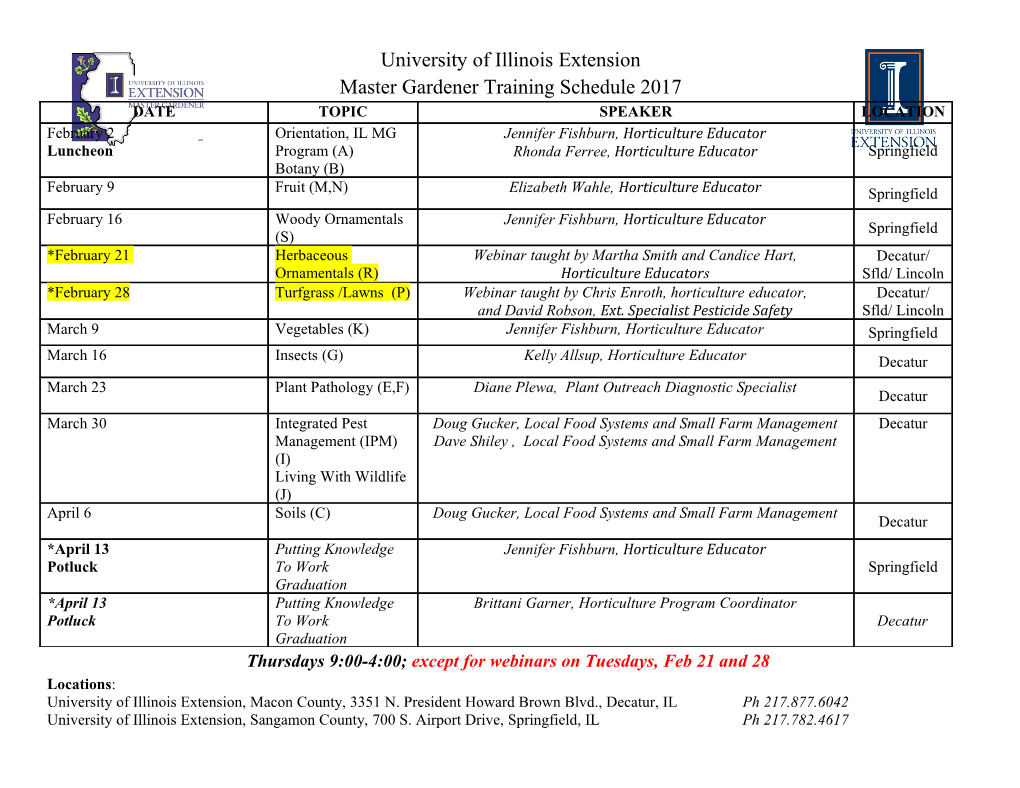
From coseismic offsets to fault-block mountains George A. Thompsona,1 and Tom Parsonsb,2 aDepartment of Geophysics, Stanford University, Stanford, CA 94305; and bUnited States Geological Survey, Menlo Park, CA 94025 Edited by Mark H. Thiemens, University of California at San Diego, La Jolla, CA, and approved August 8, 2017 (received for review June 29, 2017) In the Basin and Range extensional province of the western United pattern than the topography. Combined GPS and InSAR ob- States, coseismic offsets, under the influence of gravity, display servations (5, 6) show broad areas of uplift and subsidence in the predominantly subsidence of the basin side (fault hanging wall), with vicinity of a chain of magnitude (M) ∼ 7 earthquakes that struck comparatively little or no uplift of the mountainside (fault footwall). A central Nevada between 1915 and 1954 (7, 8). The areal extent of few decades later, geodetic measurements [GPS and interferometric vertical deformation observed over this period spans across both synthetic aperture radar (InSAR)] show broad (∼100 km) aseismic uplift basins and ranges and can be 100–200 km in width (Fig. 2). symmetrically spanning the fault zone. Finally, after millions of years However, a different deformation mode is observed during and hundreds of fault offsets, the mountain blocks display large uplift individual earthquakes (coseismic period). Highly concentrated and tilting over a breadth of only about 10 km. These sparse but subsidence (10–15 km wide) of the basins is observed (9–13) robust observations pose a problem in that the coesismic uplifts of whereas the ranges are stable, or rise very slightly (Fig. 3). the footwall are small and inadequate to raise the mountain blocks. To These observations are based on leveling surveys recorded in address this paradox we develop finite-element models subjected to Nevada before and after the 1954 M = 7.2 Fairview Peak and extensional and gravitational forces to study time-varying deforma- M = 6.5 Dixie Valley earthquakes, and in Idaho before and tion associated with normal faulting. Stretching the model under grav- after the 1983 M = 6.9 Borah Peak earthquake. Clearly, the evo- ity demonstrates that asymmetric slip via collapse of the hanging wall lution from seismic slip on faults to the final topographic signature is a natural consequence of coseismic deformation. Focused flow in the of crustal extension passes through different temporal phases. We upper mantle imposed by deformation of the lower crust localizes develop a physical hypothesis based on isostasy and crustal litho- uplift, which is predicted to take place within one to two decades after spheric rheology, and test it using finite-element models. each large earthquake. Thus, the best-preserved topographic signature of earthquakes is expected to occur early in the postseismic period. Conceptual Model Conceptually, the process begins with elastic rebound, which in rifting | finite-element modeling | earthquakes | crustal deformation | normal faulting requires a horizontal withdrawal of mass from Basin and Range the fault zone and thus an unloading of the footwall (14, 15) (Fig. 4). Isostatic forces and inflow of lower crust and upper mantle then ith its repeating series of parallel ranges rising sharply cause a postseismic upward-directed bulging, focused initially near Wabove deep valleys, the Basin and Range province is one the fault zone and later, after several decades, becomes broader. of Earth’s most distinctive terranes (Fig. 1). However, this active The basin side is repeatedly dropped with each large earthquake. ∼10–15-Ma-old (1) landscape bears little resemblance to present- Isostasy in the Earth’s crust means that its shallowest elastic day measures of deformation. During earthquakes, the basins drop parts float in the denser, hotter, and more ductile substrate below. (2), but the ranges show little or no rise. Satellite ranging [GPS, These floating masses find their equilibrium heights over time, with interferometric synthetic aperture radar (InSAR)] shows areas of that duration depending on the rheology of the substrate. Basic broad uplift and subsidence that do not directly correspond with isostatic calculations, wherein the sudden mass change after an topography. We use numerical modeling techniques constrained earthquake (1-m slip event) is treated in theoretical floating ver- with observations over three periods: coseismic (deformation tical columns, predict an upward directed force of ∼70 MPa during earthquakes), postseismic (deformation after earthquakes), and multiseismic (topography resulting from repeated earth- Significance quakes). We further constrain our models with the concept of isostasy, the gravitational equilibrium that must ultimately result Observations at different times during extensional faulting ’ from any vertical change to the Earth s surface. The addition of cycles show dramatically different deformation. Available this constraint allows us to produce a single unifying model that coseismic and postseismic observations bear little resemblance explains variations in fault-related deformation over time. to the topography of rifted zones, yet this topography is the end result of repeated earthquakes. During earthquakes, and Observations during periods decades later, there is little evidence of rift The Basin and Range province topography was built in response to flank, or range-front deformation, yet strong bending and broad crustal extension between the Sierra Nevada ranges to the uplift of these features ultimately define extended terranes. west, and the Colorado Plateau to the east. Repeated earthquakes Numerical modeling incorporating gravity and the principle of on dipping (45°–60°) faults offset crystalline bedrock ranges (fault isostatic balance predicts strong vertical forces during the first footwalls) against basins filled with sediment (fault hanging walls) decade after a significant earthquake that are preferentially (Fig. 1), meaning that roughly half of the resulting deformation is focused beneath range fronts. We conclude these forces are vertical. Cumulative slip on these faults (faults that accommodate responsible for characteristic rift topography. This hypothesis is extension are called normal faults) can be up to ∼10 km in mag- testable with intermediate period geodetic observations that nitude, and is the result of many hundreds of earthquakes. We thus are rare for extensional earthquakes. term this deformation as multiseismic. Observations and modeling results show mountain blocks that are tilted or bent upward over a Author contributions: G.A.T. and T.P. designed research, performed research, analyzed width of about 10 km (Fig. 1). Wider mountain blocks (∼20 km) data, and wrote the paper. display more bending, which requires permanent rock deformation, The authors declare no conflict of interest. probably in part expressed by myriad minor faults and joints (3, 4). This article is a PNAS Direct Submission. Deformation measured over the span of decades (postseismic 1Deceased May 12, 2017. period) using remote sensing methods shows a very different 2To whom correspondence should be addressed. Email: [email protected]. 9820–9825 | PNAS | September 12, 2017 | vol. 114 | no. 37 www.pnas.org/cgi/doi/10.1073/pnas.1711203114 Downloaded by guest on September 24, 2021 -120˚ -118˚ -116˚ 5 Warner Range Surprise Valley 0 Depth (km) 40˚ -5 0510 15 Distance (km) e g e 38˚ n a g R n a e l R a 3.4 g e B uneroded surface B’ n v i t a 2.8 h w VE 2.5:1 g i h a 2.2 N S B B’ 1.6 Elevation (km) 1.0 Syncline axis Normal Dip fault Dir. 0102030 Distance (km) Fig. 1. Characteristic basin and range topography, with narrow chains of ranges interspersed by basins. (Upper, Inset) Cross-section model of the Warner Range and its bent range front (3, 35). (Lower) The range blocks are bent as a result of repeated faulting along their fronts rather than being tilted. In this example, a single block is faced on either side by normal faults, has no significant internal faulting, and both sides are bent upward (36). applied across the narrow zone of postfaulting mass change (Fig. responsible for the signature deformation that characterizes the 4). Additionally, stress transferred from primary earthquake slip Bain and Range province and other rift zones. (16) onto the continuation of a shear zone into the deep crust can cause afterslip and additional deformation (17). Numerical Model A distinction regarding continental dip–slip faults that cause ele- A numerical model that is allowed to freely respond to forces vation changes emerges because of isostasy. Faults form and fail as a that represent our best understanding of those acting in the result of differences in the magnitudes and directions of the principal Earth’s crust can yield revealing results provided the solutions stresses in the crust, typically at a frictionally dependent angle in- are not overly guided, or overly sensitive to parameter choices. clined 30°–60° to the least compressive stress direction (18). In Gravity is the driving force acting on the crust, and the primary compressional (thrust) faulting, the least stress is vertically inclined, feature of extensional terranes is that their boundaries can ex- and the greatest stress is oriented horizontally, whereas strike–slip pand laterally, enabling the crust to collapse under its weight. We faulting regimes have horizontally directed greatest and least stresses. develop a simple finite-element model under these conditions In extensional settings,
Details
-
File Typepdf
-
Upload Time-
-
Content LanguagesEnglish
-
Upload UserAnonymous/Not logged-in
-
File Pages6 Page
-
File Size-