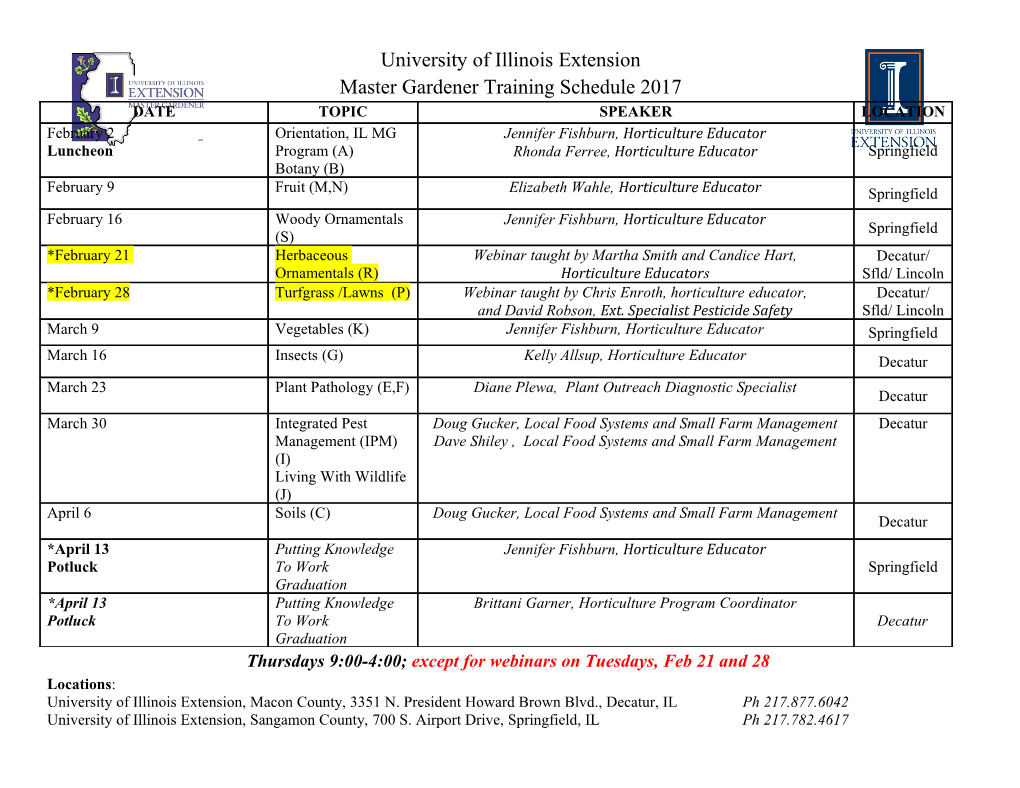
applied sciences Communication Flow Cell Coupled Dynamic Light Scattering for Real-Time Monitoring of Nanoparticle Size during Liquid Phase Bottom-Up Synthesis Nicole Meulendijks 1, Renz van Ee 1, Ralph Stevens 1, Maurice Mourad 1, Marcel Verheijen 2,3, Nils Kambly 4, Ricardo Armenta 4 and Pascal Buskens 1,5,6,* 1 The Netherlands Organisation for Applied Scientific Research (TNO), De Rondom 1, 5612 AP Eindhoven, the Netherlands; [email protected] (N.M.); [email protected] (R.v.E.); [email protected] (R.S.); [email protected] (M.M.) 2 Philips Innovation Labs, High Tech Campus 11, 5656 AE Eindhoven, the Netherlands; [email protected] or [email protected] 3 Department of Applied Physics, Eindhoven University of Technology, P.O. Box 513, 5600 MB Eindhoven, the Netherlands 4 LS Instruments AG, Passage du Cardinal 1, CH-1700 Fribourg, Switzerland; [email protected] (N.K.); [email protected] (R.A.) 5 Hasselt University, Institute for Materials Research, Inorganic and Physical Chemistry, Agoralaan Building D, B-3590 Diepenbeek, Belgium 6 Zuyd University of Applied Sciences, Nieuw Eyckholt 300, Postbus 550, 6400 AN Heerlen, the Netherlands * Correspondence: [email protected] or [email protected]; Tel.: +31-888-662-990 Received: 18 December 2017; Accepted: 10 January 2018; Published: 13 January 2018 Featured Application: A unique set-up for real-time monitoring of the size of nanoparticles during bottom-up liquid phase synthesis is presented in this article. The analysis method applied to study the size of dispersed nanoparticles during synthesis is dynamic light scattering (DLS). In contrast to conventional DLS, the DLS set-up presented in this article comprises a modulated 3D cross correlation geometry, and therefore allows accurate measurements of particle size in flow at flow rates of at least up to 17 mL·min−1. This is essential for obtaining real-time information on the size of the dispersed nanoparticles. The DLS system could be connected to reactors of various sizes using the analysis loop presented in this article, which is coupled to a flow cell in the DLS set-up. Thus, the DLS set-up presented here is suited to study the nucleation and growth of nanoparticles in dispersion, facilitates a rational scale-up, and allows intervention in the production process of nanoparticle dispersions to minimize the number of off-spec batches. Abstract: To tailor the properties of nanoparticles and nanocomposites, precise control over particle size is of vital importance. Real-time monitoring of particle size during bottom-up synthesis in liquids would allow a detailed study of particle nucleation and growth, which provides valuable insights in the mechanism of formation of the nanoparticles. Furthermore, it facilitates a rational scale-up, and would enable adequate intervention in the production process of nanoparticle dispersions to minimize the number of off-spec batches. Since real-time monitoring requires particle size measurements on dispersions in flow, conventional dynamic light scattering (DLS) techniques are not suited: they rely on single scattering and measure the Brownian motion of particles dispersed in a liquid. Here, we present a set-up that allows accurate measurements in real-time on flowing dispersions using a DLS technique based on modulated 3D cross-correlation. This technique uses two simultaneous light scattering experiments performed at the same scattering vector on the same sample volume in order to extract only the single scattering information common to both. We connected the reactor to a flow-cell in the DLS equipment using a tailor-made analysis loop, and successfully demonstrated the complete set-up through monitoring of the size of spherical silica nanoparticles during Stöber synthesis in a water-alcohol mixture starting from the molecular precursor tetraethyl orthosilicate. Appl. Sci. 2018, 8, 108; doi:10.3390/app8010108 www.mdpi.com/journal/applsci Appl. Sci. 2018, 8, 108 2 of 10 Keywords: dynamic light scattering; 3D cross correlation; real-time analysis; colloids; nanoparticle synthesis 1. Introduction To tailor the properties of nanoparticles and composite materials comprising such nanoparticles, control of the particle size is of vital importance. Nanoparticles are used in products for a wide variety of applications ranging from performance polymers to pharmaceutics, food ingredients, cosmetics and coatings and paints [1–6]. Very often, such well-defined nanoparticles are produced in bottom-up liquid phase syntheses using e.g., redox or sol-gel type reactions. A detailed study of the nucleation and growth of such particles would provide valuable information on their mechanism of formation, and could furthermore be used for process optimization and scale up. For such a study, real-time measurements of the particle size are required. For production scale synthesis, such real-time measurements could also provide data that enable rational intervention in a production process, hence minimizing the number of off-spec batches and waste. Dynamic Light Scattering (DLS) is the standard technique for determining the size of nanoparticles in the range of a few nanometers to a few microns dispersed in liquids [7,8]. It makes use of a laser to study the time dependent scattering of small particles in liquid dispersions. Since such particles undergo Brownian motion, the distance between the scattering particles in the liquid constantly changes, which results in a fluctuating scattering intensity [9]. Analysis of these fluctuations yields information about the average size and size distribution of the nanoparticles. Since in conventional DLS experiments no external flow is imposed on the dispersion, the fluctuating scattering intensity solely correlates to the diffusion of particles, and does not comprise translational information. It can thus be used to determine the diffusion coefficients of the particles, and from that the particle size distribution through use of the Stokes-Einstein equation. Consequently, such conventional DLS techniques do not allow for real-time measurements on flowing or moving dispersions. DLS measurements rely on single scattering, which means that each photon should only be scattered once in the dispersion sample before it reaches the detector. Since this requires low particle concentrations, dispersion samples typically require dilution with additional solvent which is inconvenient and may even influence the state of the dispersed colloids. To overcome this, a significant amount of research was performed in the past decade focusing on suppressing multiple scattering. It showed that multiple scattering suppression in DLS measurements can e.g., be accomplished by using two lasers operating at different wavelengths, and two detectors having distinct bandpass filters to capture scattering information from each single laser. This technique is generally referred to as two-color DLS [10]. Another more simple and robust arrangement of the beam-detector pairs is possible by displacing them symmetrically in a third dimension above and below the scattering plane, which is known as the three-dimensional (3D) cross-correlation geometry [11]. Using this geometry, two scattering experiments are performed at the same time and on the same scattering volume using two beams and two detectors. The signals seen by the detectors are cross-correlated, and only photons that are scattered once will produce correlated intensity fluctuations on both detectors. In that way, the information on single scattered photons can be extracted, and used to determine that particle size distribution in turbid dispersions. However, an important drawback of this 3D technique is that each photon detector not only receives the desired information from its correspondingly positioned laser, but also undesired information from the second laser. Thus, cross-talk occurs between the two light scattering experiments. This drawback is overcome through temporal isolation of the two beam-detector pairs in modulated 3D cross-correlation DLS. Appl. Sci. 2018, 8, 108 3 of 10 Real-time DLS measurements moreover are challenging since a flow is imposed on the dispersion in laboratory and pilot-scale reactors, e.g., through stirring or pumping. Accurate particle sizing using DLS usually necessitates a resting fluid in the measured sample [12]. In actively mixed fluids, the diffusion is overlaid by a turbulent convection which prohibits diffusion measurements. Here, we demonstrate a set-up based on modulated 3D cross-correlation DLS, which is capable of separating the scattering information resulting from diffusional and translational motion of particles, and consequently allows flow cell coupled measurements on flowing nanoparticle dispersions [13]. In this setup, we pump a particle dispersion from the reactor through an analysis loop comprising a flow cell, and back into the reactor. We successfully validated the set-up presented in this article through monitoring of the size of spherical silica (SiO2) nanoparticles during Stöber synthesis starting from the molecular precursor tetraethyl orthosilicate (TEOS). Conventional off-line DLS on non-moving liquid dispersions using a state of art DLS instrument, and transmission electron microscopy (TEM) are used to validate the particle size information obtained with our newly developed flow cell coupled DLS instrument. 2. Materials and Methods 2.1. Materials Ethanol (EtOH) was obtained from Biosolve (Valkenswaard, the Netherlands) and used without further purification. Tetraethyl
Details
-
File Typepdf
-
Upload Time-
-
Content LanguagesEnglish
-
Upload UserAnonymous/Not logged-in
-
File Pages10 Page
-
File Size-