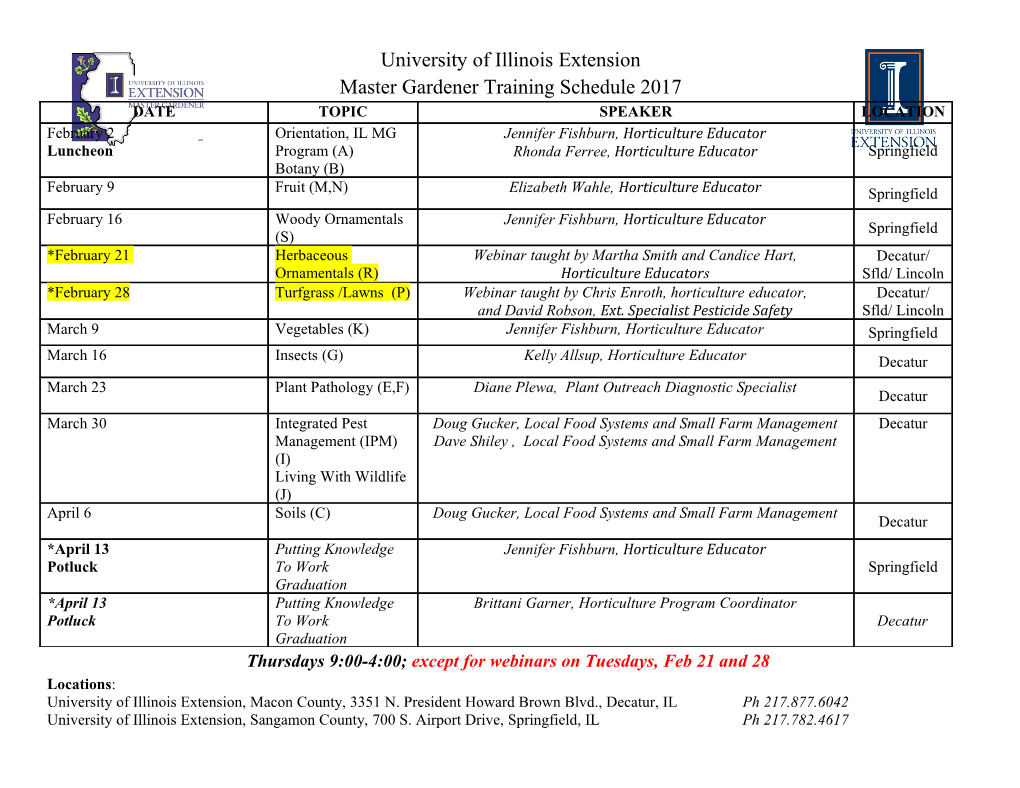
Brittle deformation adjacent to the Awatere strike-slip fault in New Zealand: Faulting patterns, scaling relationships, and displacement partitioning Timothy A. Little Department of Geology, Victoria University of Wellington, P.O. Box 600, Wellington, New Zealand ABSTRACT is a poor index of slip sense on the mature (Chester et al., 1993; Cowie and Scholz, fault. Close to the Awatere fault, dextral- 1992). Brittle fabrics in fault zones also may On the coast of South Island, New Zea- reverse faults are synthetic to that mature contain a record of early displacement in- land, the active dextral-reverse Awatere structure. Their disposition indicates incre- crements along faults with complex slip his- fault has <2 km finite dextral slip and in- mental compression acting '45&–50& clock- tories. Recent papers have cited aspects of itiated <4 Ma. Fault spacing along '2km wise to the strike of the Awatere fault. These the fractal nature of fault populations to of coastal sea cliffs decreases from >22 m to youngest faults mirror the slip kinematics constrain models on fault growth and fault- <0.5 m as one approaches the fault from the of the adjacent mature fault. Their attitude ing-related strain, but field data on scaling north. The pattern of fault attitudes and is controlled apparently by local kinematics, relationships for natural faults are sparse slip directions is independent of scale. A not by far-field stress orientations. (Cowie and Scholz, 1993; Gillespie et al., power-law relationship between slip and cu- 1993; Scholz et al., 1993). mulative frequency of faults has an expo- INTRODUCTION The active Marlborough fault system in nent of '0.8 for this linear outcrop sample. the northeastern part of the South Island of Near the Awatere fault, faulting-related Displacement along major faults is not New Zealand splays northeastward from the strains are large, reflecting partitioning of confined to a single plane, but is distributed Alpine fault to comprise an '100-km-wide slip into late-stage faults near the core of into the adjacent rock volume by arrays of zone of distributed deformation between the active fault; farther away, they are very subsidiary faults (e.g., Tchalenko, 1970; the Pacific and Australian plates (Fig. 1). small, where most of the small-scale fault- Wojtal, 1986). Structures bordering faults Pliocene–Holocene motion of the Pacific ing is relict. Strain softening led to local- preserve a record of deformation mecha- plate relative to the Australian plate trends ization of slip into (and near) the fault’s nisms and stress distributions and may pro- nearly west (DeMets et al., 1990), resulting core and was assisted by suprahydrostatic vide insight into the fault growth process in transpression across the Marlborough pore-fluid pressure gradients, but evidence for fault-orthogonal compression and ex- treme fault zone weakness is lacking. Two styles of gouge on faults both have linear scaling ratios relating gouge thickness to fi- nite slip. Different ratios for each may in- dicate coseismic and aseismic gouge gener- ation processes acting at crustal depths of 1–2 km. Early tensile cracking of pebbles is interpreted to have occurred in the process zone of the Awatere fault as it propagated upward as a mode III crack. If so, the fault has a process zone width/finite slip ratio of >1.5, consistent with an elastic-plastic model of fault growth. Younger domino- style oblique-normal faults resulted in oblique extension of the Awatere fault zone, with mean s1 oriented '30& clockwise from its strike. Transtension may have been caused by divergence between rotating, crustal-scale fault blocks, the result of a Figure 1. Simplified tectonic map of the New Zealand region showing relative motion of mode II fault tip to the east, which caused a Pacific plate relative to Australian plate, the actively deforming plate boundary zone (stip- local rotation of stress trajectories. The pled), the Alpine fault, and the Marlborough faults. Adapted from Walcott (1989) and early faults are relict, and their kinematics DeMets et al. (1990). GSA Bulletin; November 1995; v. 107; no. 11; p. 1255–1271; 16 figures. 1255 Downloaded from http://pubs.geoscienceworld.org/gsa/gsabulletin/article-pdf/107/11/1255/3382102/i0016-7606-107-11-1255.pdf by guest on 29 September 2021 T. A. LITTLE biozones with a detailed magnetostratig- raphy established for the Awatere basin (Roberts et al., 1994) indicates that the rocks at White Bluffs were deposited ca. 7–4 Ma. On both sides of the fault, strata dip on average '108 to the northeast (Figs. 3a and 3b) and are at least 600 m thick. Upright folds with gently northeast plunging hinges warp the Neogene se- quence. Beds dip on average '108 to the southeast or south, but are variably tilted in detail (Fig. 3c). Detailed mapping near the coast indi- cates that the Awatere fault strikes 063, dips 708–908 to the northwest, and defines a gentle releasing bend with respect to the mean 055 strike of more inland parts of the fault (Fig. 2). A series of stream chan- nels near the coast are offset dextrally Figure 2. Tectonic map of the Marlborough faults, South Island, New Zealand. For 150–200 m by the fault. South of the active location, see Figure 1. Active fault traces and estimates of fault slip rates (mm/yr, in part of the Awatere fault, an inactive splay parentheses) compiled from Van Dissen and Yeats (1991) and Kneupfer (1992). Direction of that fault strikes slightly clockwise from of maximum incremental horizontal shortening (PHS) from geodetic surveys shown with the active trace (Lensen, 1963). Its trace is ball-and-bar symbol and taken from Bibby (1976, 1981), Lamb and Bibby (1989), and defined topographically by a range of hills Walcott (1984); PHS inferred from earthquake focal mechanism solutions (double arrows), on its northern side, but is concealed be- from Arabasz and Robinson (1976), and Anderson et al. (1993). Velocity of Pacific plate neath a flight of Holocene alluvial terraces with respect to Australian plate near Figure 3 calculated from Nuvel-1 pole of DeMets et (Eden, 1983). al. (1992). Abbreviations nb 5 Nina Brook and bl 5 Blarich Stream. Over 260 m high, the White Bluffs provide superb exposure of faults and other brittle faults, which have undergone dextral-reverse from the active Awatere fault. This data has structures on the north side of the active slip during late Neogene time. Pebble rind implications for (1) displacement-gouge trace of the Awatere fault. The distribution dating of offset terraces at two sites along thickness and displacement-frequency scal- and orientation of faults and fault rocks the Awatere fault (Fig. 2) indicates Holo- ing relationships in natural fault popula- were recorded in a compass-pace/detailed cene dextral-slip rates of '4–7 mm/yr and tions, (2) the nature and width of zones of mapping traverse along the north- to north- horizontal/vertical displacement ratios .10 small-scale faulting bordering major upper west-trending coastal bluffs. These comprise (Kneupfer, 1992). In the upper Awatere crustal strike-slip faults and variation in time an essentially linear sample of the fault pop- Valley, Silberling et al. (1988) inferred a fi- of the directions of principal stress/incre- ulation at high angle to the strike of the nite dextral displacement of '16 km for the mental strain within such zones, (3) the Awatere fault. Data were projected (by Awatere fault from offset of a near-vertical kinematics of faulting in zones of oblique computer) onto a transect normal to the melange unit within basement rocks of the extension, and (4) models for brittle defor- northeast strike of the Awatere fault. A cu- Mesozoic Torlesse terrane. Farther north- mation and fault-zone evolution associated mulative width of 250 m was covered by east in the lower Awatere Valley, Little with the rupture and finite growth of large slumps or colluvium, and 750 m occurred (1994) inferred .32 km of dextral slip from faults. within a reach of inaccessible cliffs. In the the minimum offset of a marker line defined remaining 2 km of composite exposure, 307 by the intersection of bedding and a facies WHITE BLUFFS EXPOSURE OF THE faults were observed and measured in detail. boundary in a late Miocene (ca. 6–8 Ma) AWATERE FAULT ZONE Figure 4 summarizes the types of observa- marine basin transected by the fault (Fig. 2, tions made. A computer program calculated stipple pattern). Near the coast, a sequence of late Mio- displacements for each fault, based on offset The study region for this paper is located cene–early Pliocene marine conglomerate of bedding on outcrops and slip-lineation along the active trace of the Awatere fault and mudstone occurs on both sides of the pitch (Little, in press). on the northeast coast of the South Island Awatere fault (Fig. 3). Planktonic and (Fig. 3). North of the fault, Neogene rocks benthic foraminiferal assemblages in 20 INCEPTION AGE OF AWATERE are superbly exposed in sea cliffs, allowing samples from both sides of the fault are FAULT SEGMENTS detailed cross-sectional study of .300 small- late Tongaporutuan to possibly earliest er-scale faults. In this paper, I describe the Kapitean (New Zealand stages, late Mio- Inception of the Awatere fault is inferred pattern, distribution, and sequential devel- cene) and, at the highest preserved levels, to postdate the 6.5–8 Ma age (Roberts et al., opment of tensile fractures and faults vary- early Pliocene. The assemblages are char- 1994) of the late Tongaporutuan coastal sec- ing in scale over several orders of magnitude acteristic of outer shelf to upper bathyal tion, as these marine rocks were deposited and quantify faulting-related displacement paleodepths (P.
Details
-
File Typepdf
-
Upload Time-
-
Content LanguagesEnglish
-
Upload UserAnonymous/Not logged-in
-
File Pages17 Page
-
File Size-