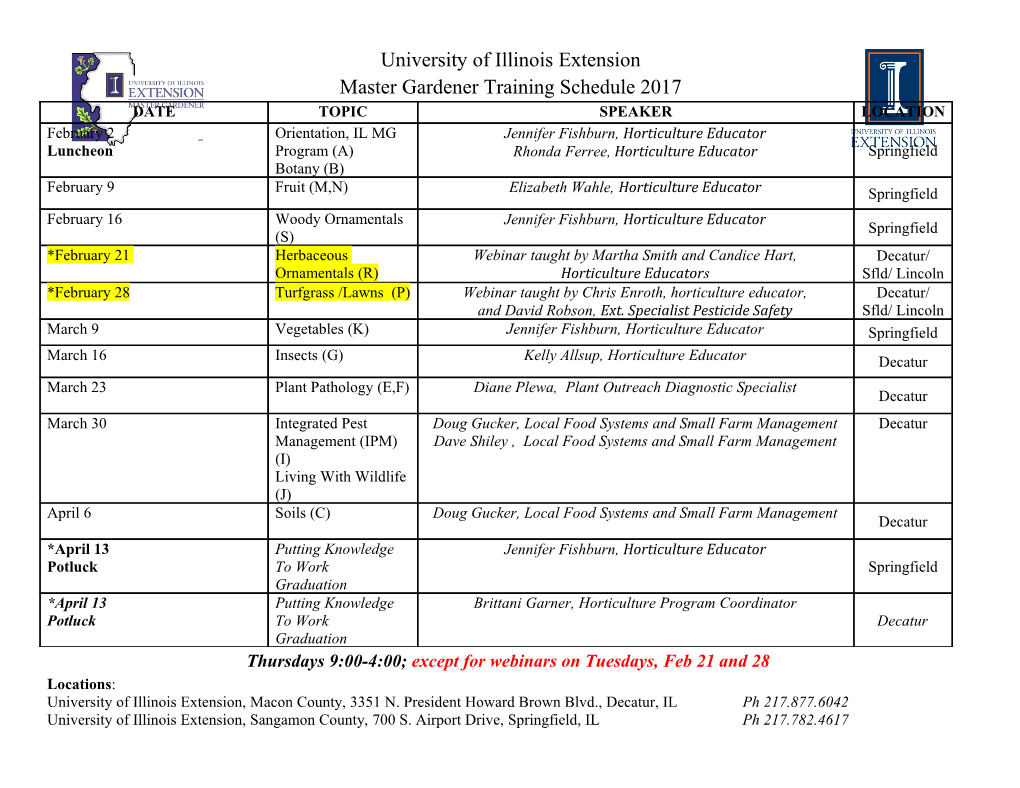
Doppler Imaging of Starspots: Spectral Diagnostics. A Case Study of the RS CVn Star σ Geminorum Dissertation zur Erlangung des Doktorgrades der Mathematisch-Naturwissenschaftlichen Fakultaten¨ der Georg-August-Universitat¨ zu Gottingen¨ vorgelegt von Alina A. Semenova aus Pavlograd/Ukraine Gottingen¨ 2006 D7 Referent: Prof. Dr. Stefan Dreizler Korreferent: Prof. Dr. Sami K. Solanki Tag der mundlichen¨ Prufung:¨ Dedicated to my sister... Contents 1 Introduction 3 2 Doppler Imaging Technique 5 2.1 Starspots: history, properties, comparison with sunspots . ....... 5 2.2 Basics of the Doppler imaging technique for mapping of stellar surfaces . 6 2.3 Necessary conditions for Doppler imaging . ............... 10 2.4 Basics of the inverse problem . ....................... 12 2.4.1 Calculation of Doppler shifted spectral line profiles . ....... 12 2.4.2 The inverse problem . ....................... 14 2.4.3 The Bayesian approach and codes based on it ........... 15 2.4.4 The Occamian approach . ................... 16 2.5 Doppler imaging code based on the Occamian approach . ....... 19 2.5.1 Calculation of number densities of atoms and molecules . 19 2.5.2 Calculation of local line profiles . ............... 19 2.5.3 Limb darkening coefficients . ................... 20 2.5.4 Surface grid and geometry . ................... 20 2.5.5 Inversions .............................. 21 3 Doppler Imaging of Sigma Geminorum 23 3.1 RS CVn binary system σ Geminorum ................... 23 3.1.1 General information, orbital elements and photometric properties 23 3.1.2 Spectroscopic properties . ................... 25 3.1.3 The secondary component . ................... 26 3.2 Observations . .............................. 26 3.2.1 Nordic Optical Telescope . ................... 26 3.2.2 Telescope Bernard Lyot . ................... 27 3.2.3 Data description . ....................... 27 3.3 Studying of the physical parameters of σ Geminorum ........... 33 3.3.1 Surface gravity . ....................... 34 3.3.2 Turbulent motions: microturbulence and macroturbulence . 36 3.3.3 Effective temperature . ....................... 44 3.3.4 Inclination . ....................... 50 3.3.5 Rotational velocity . ....................... 54 3.4 Influence of the atomic lines selected for DI on the reconstructions. 57 3.4.1 4 atomic lines: Fe I 6173 A,˚ Ni I 6175 A,˚ Ni I 6177 A,˚ Fe I 6180 A59˚ 3.4.2 2 atomic lines: Fe I 6430.85 A˚ and Ca I 6439.08 A.........˚ 61 1 Contents 3.4.3 3 lines of Fe I . ....................... 64 3.4.4 2 lines of Ni I . ....................... 64 3.4.5 Doppler Imaging with Mean Line Profile. ........... 65 3.5 Summary and conclusions . ....................... 72 4 Results of Surface Imaging of σ Gem for the period of 1998-2001. 75 4.1 February 1998 . .............................. 75 4.1.1 Reconstruction with 4 atomic lines . ............... 75 4.1.2 Reconstruction with 6 atomic lines . ............... 75 4.2 February 2000 . .............................. 80 4.2.1 Reconstruction with 4 atomic lines . ............... 80 4.2.2 Reconstruction with 6 atomic lines . ............... 80 4.3 December 2000 . .............................. 85 4.3.1 Reconstruction with 4 atomic lines . ............... 85 4.4 December 2001 . .............................. 85 4.5 The evolution of spots during 1998 - 2001 . ............... 89 4.6 Summary and conclusions . ....................... 92 5 Summary and outlook 95 A The list of Fe I lines and their weights used for extracting of LSD-profile 97 Bibliography 103 Aknowledgement 109 Lebenslauf 111 2 1 Introduction The study of cool stars is an important topic in astrophysics. Stars are considered to be cool stars with photospheric temperatures TPh < 7000 K and spectral types later than A, i.e. stars with an outer convection zone. Convection combined with high stellar rotation leads to the appearance of a dynamo mechanism which converts the mechanical energy of convective rotation and motion into magnetic energy. Sighns of stellar activity (for example, spots or plages), as a result of magnetic dynamo, appear on the surface. The activity level depends mainly on the rotation velocity: the faster a star rotates, the more active it is. In the Hertzsprung-Russel Diagram, where the spectral class or the surface temperature is given on the x-axis and the luminosity on the y-axis (Fig. 1.1), cool stars occupy the right side (hatched region). A typical example of a star from this class is our Sun. The Sun is a normal star – a yellow dwarf – from the middle-lower part of the main sequence with the spectral class G2 and the effective temperature 5777 K. Because of its rather close distance to the Figure 1.1: Herzsprung-Russel Diagram. The hatched section on the top right marks the location of cool stars. 3 1 Introduction Earth (approximately 1.5 × 108 km), it was the first star where activity on the surface was observed. A first report about sunspots was published on 9th of March, 1611 by David Febricius not long after the telescope had been brought into astronomy in 1609 by Galileo Galilei. Although observational techniques have evolved much since that time, the Sun is still the only star where details of the topographic structure can be observed directly (except α Ori= Betelgeuse, whose surface has been directly imaged with the Faint Object Cam- era on the Hubble Space Telescope in March 1995). Even using the largest telescopes, one cannot resolve the surface structure of other stars. It is only possible to observe the surface-integrated radiation emitted from the visible stellar disk, e.g. the radiation flux. However, it is known that the activity phenomena like those on the Sun also occur on other stars. The underlying mechanisms of activity phenomena in stars of different spectral classes are of different origin. Single and well separated binary stars the cause of activity is stress- ing of the magnetic field by convective motions. Members of close binary systems, such as the RS Canum Venaticorum systems, have an additional mechanism — the interaction of magnetic fields with the magnetospheres of the companion star. In pre-main sequence stars magnetic fields can also interact with the accretion disk. Obviously, investigations of physical processes on the Sun help in understanding of structuring and evolution of stellar atmospheres. Many of the physical processes assumed to occur on stars are known from the Sun. However, the study of only one star is not enough if one wants to be able to predict how the magnetic activity of a star with a given spectral type, temperature, mass, chemical composition and rotational period will evolve in time until its end. 4 2 Doppler Imaging Technique 2.1 Starspots: history, properties, comparison with sunspots One of the visible signs of a magnetic field on a star is the presence of cool features (e.g. spots) on its surface. First indirect observations of stellar spots were made by Hertzsprung (1924) without knowing the origin of this phenomenon: a different brightness of the same star was observed during one observing night. Hertzsprung supposed that it was an aster- oid which fell down on the star and caused the change in brightness. Later, in 1950, the International Astronomical Union (IAU) classified all stars with similar behaviour to the new class of periodically variable pulsars. However, now we know that in this case we are dealing with stars covered with spots similar to those observed on the Sun. The existence of magnetic activity on the surface and in the atmosphere of several types of stars is today generally accepted and has been investigated by several methods. For example, measuring the chromospheric excess emission can indicate a presence of magnetic activity Wilson (1978). Ca II H and K emission and X-ray emission serve as probes of the high chromospheric and coronal activity. The presence of cool spots on the stellar surface is indicated by the periodic variation of the visible light during a star’s rotation. To specify this activity on the rapidly rotating stars in more detail one can analyse time-series of spectral line profiles Rice (1996). This method is called Doppler Imaging and it will be described later in this chapter. At present, we know 8 groups of stars which are believed to have spots of magnetic origin on their surfaces (Berdyugina 2005). There are: 1. red dwarfs and BY Draconis stars (main sequence star of spectral type M or late K with the mass range from 0.08M to 0.5M); 2. solar-type stars (similar to the Sun in mass and evolutionary state stars on the lower main-sequence with a spectral type from F7 to K2); 3. T Tauri stars (young, less than 10 million years, pre-main-sequence stars of about 1M); 4. FK Com stars (late-type giants of G to K spectral type with a very short rotational periods of only a few days); 5. RC CV type stars (close detached binaries with a more massive primary component, which is G-K spectral type giant and the secondary component, which is a G-M spectral type subgiant or dwarf); 5 2 Doppler Imaging Technique 6. W Uma stars (eclipsing binaries, which consist of two solar-type components sur- rounded by a common envelope and have a periods of 5 − 20 h); 7. Algols (eclipsing binaries with a hot, main-sequence primary component of B-F spectral class and a cool evolved, less massive secondary component, which is a G or K subgiant). Although stellar spots are believed to be similar to sunspots, there are two major differences between them. First, all of the detected stellar spots have sizes much lager then sunspots. First observations of photometric variability predicted stellarspots 100 times bigger than sunspots Hall (1972), Rodono et al. (1986). This can be a result of a larger amount of magnetic flux on active stars or the low resolution of the detection techniques: it is possible that a group of small spots is seen as one big spot.
Details
-
File Typepdf
-
Upload Time-
-
Content LanguagesEnglish
-
Upload UserAnonymous/Not logged-in
-
File Pages115 Page
-
File Size-