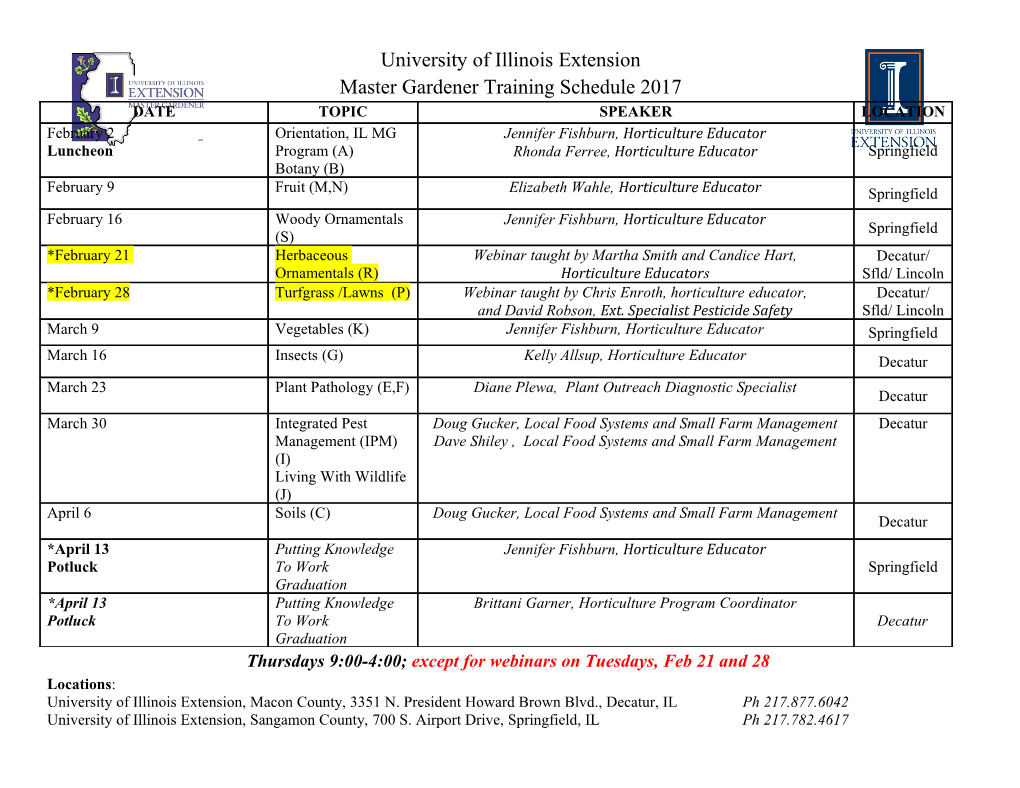
Publications of the Astronomical Society of Australia (PASA), Vol. 32, e018, 7 pages (2015). C Astronomical Society of Australia 2015; published by Cambridge University Press. doi:10.1017/pasa.2015.18 Central Compact Objects in Kes 79 and RCW 103 as ‘Hidden’ Magnetars with Crustal Activity S. B. Popov1,4,A.A.Kaurov2 and A. D. Kaminker3 1Sternberg Astronomical Institute, Lomonosov Moscow State University, Universitetskii pr. 13, 119991 Moscow, Russia 2Department of Astronomy and Astrophysics, The University of Chicago, Chicago, IL 60637, USA 3Ioffe Physical-Technical Institute, Politekhnicheskaya 26, Saint Petersburg 194021, Russia Email: [email protected] (Received January 30, 2015; Accepted April 13, 2015) Abstract We propose that observations of ‘hidden’ magnetars in central compact objects can be used to probe crustal activity of neutron stars with large internal magnetic fields. Estimates based on calculations by Perna & Pons, Pons & Rea and Kaminker et al. suggest that central compact objects, which are proposed to be ‘hidden’ magnetars, must demonstrate flux variations on the time scale of months–years. However, the most prominent candidate for the ‘hidden’ magnetars — CXO J1852.6+0040 in Kes 79 — shows constant (within error bars) flux. This can be interpreted by lower variable crustal activity than in typical magnetars. Alternatively, CXO J1852.6+0040 can be in a high state of variable activity during the whole period of observations. Then we consider the source 1E161348−5055 in RCW103 as another candidate. Employing a simple 2D-modelling we argue that properties of the source can be explained by the crustal activity of the magnetar type. Thus, this object may be supplemented for the three known candidates for the ‘hidden’ magnetars among central compact objects discussed in literature. Keywords: stars:individual (Kes 79, RCW 103) – stars:magnetars – stars:neutron. 1 INTRODUCTION useful to observe magnetars without crust activity (or with completely stable crust), but with evidences of active magne- Magnetars — neutron stars (NSs) whose activity is related to tospheric events, and magnetars with definitely suppressed dissipation of the magnetic field energy — have many differ- magnetospheres, but with signs of active crustal processes. ent observational appearances (see recent reviews Mereghetti Then we would be able to conclude what triggers different 2008; Rea & Esposito 2011). Strong bursts observed in hard types of activity: processes in the magnetosphere and/or in X-rays are the most spectacular manifestations of this activ- the crust. ity. What triggers magnetar bursts and long term outbursts1 Objects of the first kind are extremely difficult to identify, is not known. Two main and probably causally associated even though bare strange stars potentially can exist without a approaches are discussed (see for example, Levin & Lyu- crust, (see e.g., Page & Usov 2002 and references therein). As tikov 2012 and references therein). Bursts are either related for the second kind of objects, three central compact objects to crust cracks of some kind, or occur due to (possibly ensu- (CCOs,seeareviewbydeLuca2008) are observed, which ing) magnetospheric activity (e.g., Parfrey, Beloborodov, & are believed to be so-called ‘hidden’ magnetars, for example Hui 2013; Beloborodov & Levin 2014;Link2014). like CXO J1852.6+0040 in Kes 79. The hypothesis of their Magnetars activity is not uniform in time. There are pe- suppressed magnetosphere is mainly based on the analysis riods of high state of activity (outbursts), and quiescent pe- of their thermal emission: pulse profiles of the X-ray light riods. The rate of energy release on long time scale is most curves and a high pulse fraction, which requires magnetar- likely driven by crustal processes, including field evolution scale fields in the crust (e.g., Shabaltas & Lai 2012;Vigano` in the crust. In order to compare relative importance and in- & Pons 2012; Perna et al. 2013; Bogdanov 2014). terplay of magnetospheric and crustal processes, it would be The idea of ‘hidden’ magnetars dates back to 1999, when the term was first proposed by Geppert, Page, & Zannias 1We use the term burst for a single event, and outburst for a long-term (1999). Strong fall-back after a supernova explosion (Cheva- emission enhancement during which a few or many bursts can be observed. lier 1989) can lead to formation of an envelope which screens 1 Downloaded from https://www.cambridge.org/core. IP address: 170.106.40.40, on 25 Sep 2021 at 18:23:23, subject to the Cambridge Core terms of use, available at https://www.cambridge.org/core/terms. https://doi.org/10.1017/pasa.2015.18 2 Popov et al. the magnetic field. Therefore, for an external observer the NS is visible as a source with low dipole field B ∼ 1010 G. Cal- Chandra −4 102 culations show that an envelope with a mass ∼10 M is Swift enough to screen the field (see for example, Bernal, Page, XMM &Lee2013). However, these sources might be different from low-field magnetars (see Rea et al. 2014 and references therein), for which strong external multipoles are detected (Tiengo et al. 2013). 0 One can expect that observational features of ‘hidden’ L/L 10 magnetars are the following: (i) non-uniform surface temper- ature distribution, for instance, relatively small hot spots on the surface of an NS, (ii) large pulse fractions, (iii) flux vari- ations on a time scale of months-to-years, which is typical for magnetars. In reality, candidates for the ‘hidden’ mag- netars can manifest only some of these features. Anyway, ‘hidden’ magnetars can be important to probe initial prop- 1 erties of highly magnetized NSs (and, probably, the origin 0 1 2 3 4 5 6 7 8 of huge magnetic field). For instance, their spin periods stay Time [yr] almost constant for several kyrs due to low spin-down rate (Popov 2013), and therefore initial rotation rate is ‘frozen’ Figure 1. Simulated thermal X-ray luminosities L as a function of time t in these objects. In the following sections, we present simple versus observational X-ray data on 1E 161348−5055 in the time interval estimates which demonstrate that ‘hidden’ magnetars can be from 1999 September to 2007 July (De Luca et al. 2008). Time zero cor- responds to the first observation of the outburst. Observational fluxes and also used to probe properties of large magnetic fields in the calculated luminosities are normalized to the flux F0 and luminosity L0 at NS crust. the zero moment (see text). Three light curves correspond to three different ρ ≤ ρ ≤ ρ heaters (see Table 1) in the NS crust located in layers 1 2 within solid angles 0 around a symmetry axis (see text). Long dashed curve cor- 2 ANALYSIS AND ESTIMATES responds to the model (a) in Table 1; dotted and solid lines — to models (b) and (c), respectively. All heaters start energy release at the same moment and In this section, we produce simple estimates of crustal ac- = continue for 120 d. The age of the NS at this moment is t0 2 kyr. Chandra tivity for different CCOs, which potentially can be ‘hidden’ data is shown with empty circles, XMM–Newton data — with diamonds, magnetars, and present numerical model for the case of the and Swift data — with filled triangles (error bars are disregarded). CCO in RCW 103. 2.1. Expected rate of CCO activity the hot spots on the surface can form and move in respect We start with estimates based on results by Perna & Pons to their relatively constant position in quiet state. The pulse (2011) and Pons & Rea (2012). These authors provide calcu- profile then might become more complicated, and the pulsed lations of the rate and power of energy release events (ERE) fraction can increase, as well as decrease compared to the in the crust of NSs, and then estimate their surface luminosity. quiet state. The X-ray spectra in such states can be fitted by a Since we consider sources similar to CXO J1852.6+0040 sum of two blackbodies with temperatures corresponding to in Kes 79 (hereafter just Kes 79), typical ages of interest hot spots or the hot spot and the rest surface without activity. are about few kyrs. In terms of Perna & Pons (2011), these Now we make estimates based on calculations by sources are between ‘young’ and ‘middle age’ NSs. Depend- Kaminker et al. (2014, hereafter Paper I). These authors nu- ing on the parameter which characterises the fatigue limit of merically modelled surface and neutrino luminosity of an material subjected by magnetic stresses, these authors predict NS as a result of a rather long (tens of kyrs) ERE in the that for objects of this age EREs happen approximately once crust. The key parameter is the heat rate H [erg cm−3 s−1]. in a few years, with bimodal distribution of typical released Paper I shows that the surface luminosity nearly saturates for ∼ 40 41 ∼ 43 44 20 −3 −1 energies Etot 10 –10 ergs and Etot 10 –10 ergs. H 10 erg cm s . Here, we estimate Etot of an ERE Relative fraction of these two ERE types can change by a with this heat rate. factor 2–3 in favour of one or another. First, we need to estimate the total volume in which energy According to Pons & Rea (2012), an ERE with is released. In Paper I, the authors used the thickness of the ∼ × 43 ∼ Etot few 10 ergs provides a surface luminosity above layer where energy is released 100 m. Pons & Rea (2012) 2 × 1034 erg s−1 for ∼100 d (their Figure 1). We expect that used the layer with thickness ∼200 m. The surface area of the more than 10% of time the source is in a high state with region is typically given in terms of its angular size.
Details
-
File Typepdf
-
Upload Time-
-
Content LanguagesEnglish
-
Upload UserAnonymous/Not logged-in
-
File Pages7 Page
-
File Size-