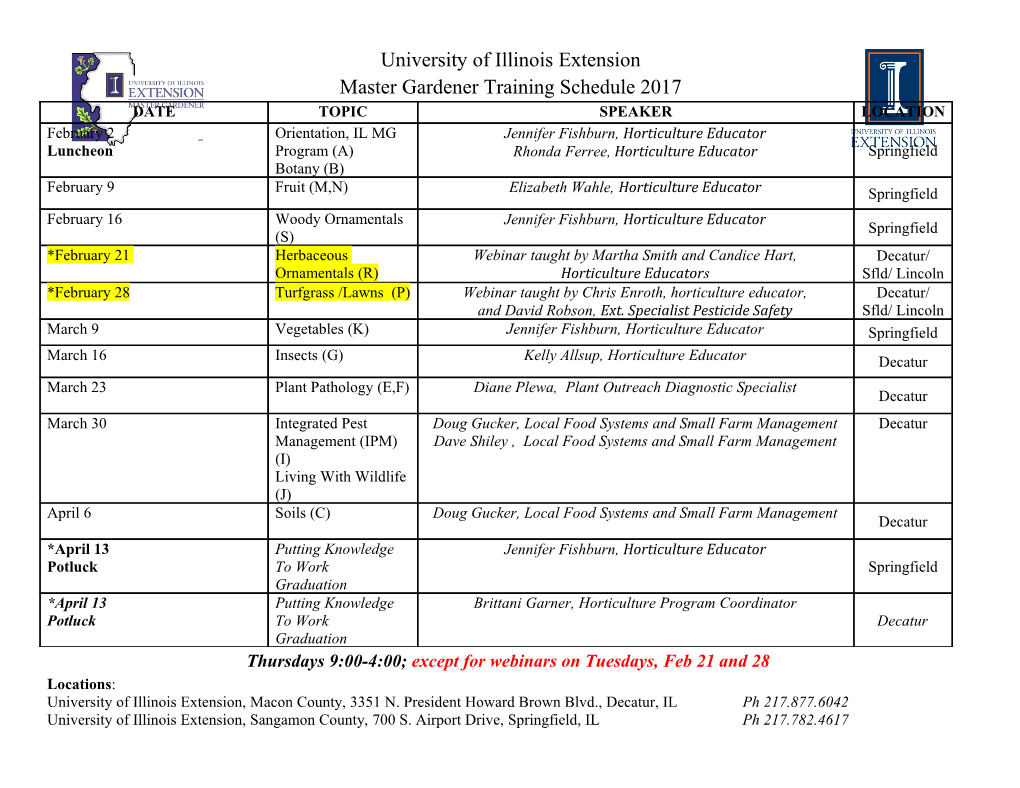
www.nature.com/scientificreports OPEN The role of dislocation‑solute interactions on the creep behaviour of binary Mg–RE alloys Jing Li1, Jialin Wu1, Li Jin1*, Mert Celikin2, Fenghua Wang1, Shuai Dong1 & Jie Dong1 The efect of dislocation‑RE atoms interactions on the creep behaviour has been studied via creep testing and HAADF‑STEM analysis of two extruded alloys; Mg–0.5Ce and Mg–2Gd (wt%). Almost no Ce atoms are detected in the Mg matrix due to the low solid solubility and faster difusion rate in as‑extruded condition. However, Gd solute segregations are observed along dislocations and hexagonal dislocation patterns. Such segregations can not only pin the dislocation motion and enhance the creep strengthening via dislocation patterns, but also lead to dynamic precipitation. Thus, combing with the stress exponent values, the transition of creep mechanism between Mg–0.5Ce alloys and Mg–2Gd alloys has been found and dislocation‑Gd atoms interactions are determined to be the main factor for superior creep resistance of Mg–2Gd alloys. Rare earth (RE) elements are intentionally added to pure Mg to modify the mechanical properties1–4. If the RE concentration is lower than its solid solubility in pure Mg, the strengthening efect ordinarily comes from the solid solution strengthening, which is conventionally attributed to the elastic interactions between dislocations and local lattice strain caused by RE atoms 5. However, at high temperatures, the large diferences in the atomic radii between RE and Mg atoms can also lead to solute segregation around dislocation cores. Such solute clusters or orderings can exert a drag force on the gliding dislocations, slow down the dislocation motion and thus tailor the macroscopic properties of Mg alloys6–9. One typical example regarding such interactions is the Portevin–Le Chatelier (PLC) efect10. Within particular temperature and strain rate ranges, mobile dislocations can be con- tinuously pinned and unpinned by RE solute atmospheres and ultimately exhibiting serrations in the fow curve. Zhu et al.11 have reported that the PLC efect can not only lead to the negative sensitivity of stress to strain rate but also a plateau in the temperature dependent yield and ultimate strength. Moreover, dislocation substructures can serve as templates for the segregation of RE atoms at high temperatures12. Li et al.13 discovered the hexago- nal patterning Gd nanofbers in extruded Mg–Gd binary alloys and illustrated that these nano structures can potentially modify the mechanical performance by tunning the work hardening behaviour. Terefore, it is of importance to pay attention to the interactions between dislocations and RE atoms as well as their efect on the macroscopic properties, particularly for the plastic deformation at high temperatures. Limited creep resistance has been regarded as one of the principal hurdles which are restricting the wide- spread applications of Mg alloys 14,15. One critical strategy enhancing the creep performance is to alloy Mg with RE elements. To date, solid solution and dynamic precipitation strengthening, if possible, are the two main hard- ening mechanisms which are responsible for the appealing creep properties 16–18. Mo et al.19 also tried to relate the superior high temperature behavior of binary Mg–RE solid solutions to the short-range order strengthening efect. However, the nature of interactions between dislocations and RE solutes is still ambiguous despite the studies available that have successfully used modelling to shed light into the efects of RE elements20,21. Tere is a lack of experimental data on dislocation-RE solute interactions during creep as it is challenging to isolate the efect of solutes only where grain size and precipitates are important factors as well. In this study, we investigated the dislocation-solute interactions in two binary Mg–0.5Ce and Mg–2Gd (wt%) alloys crept at 200 °C, using high-angle annular dark-feld scanning transmission electron microscopy (HAADF-STEM) technique to obtain atomic scale information. Ce and Gd elements are chosen here because they are the most common REs and have diferent solid solubility limits in pure Mg, i.e., 0.74 wt% and 3.82 wt%, respectively, at 200 °C20. Te results might provide valuable information for better understanding of strengthening mechanism for solid solution Mg–RE alloys with enhanced creep resistance and shed light for creep resistant Mg alloy design. 1National Engineering Research Center of Light Alloy Net Forming and State Key Laboratory of Metal Matrix Composite, School of Materials Science and Engineering, Shanghai Jiao Tong University, Shanghai 200240, China. 2I-Form Advanced Manufacturing Research Centre, School of Mechanical and Materials Engineering, University College Dublin, Belfeld, Dublin 4, Ireland. *email: [email protected] Scientifc Reports | (2021) 11:2860 | https://doi.org/10.1038/s41598-021-82517-5 1 Vol.:(0123456789) www.nature.com/scientificreports/ Figure 1. Microstructure of extruded Mg–0.5Ce (the frst row) and Mg–2Gd alloys (the second row) before creep tests: (a,d) EBSD orientation maps, (b,e) KAM images, (c,f) SEM images and EDS analysis. Methods Mg–0.5Ce and Mg–2Gd alloys ingots were solution treated at 520 °C for 8 h, followed by quenching into water, and then extruded at 350 °C. Dog-bone tensile samples (with a gage length of 15 mm and cross-sectional dimen- sion of 3.5 × 2.2 mm2) and fat tensile creep samples (with a gage length of 25 mm and cross-sectional dimension of 6 × 2 mm2), in accordance with the ASTM-E21 and ASTM-E139 standards, respectively, were machined out of the two as-extruded bars along the extrusion direction (ED). High temperature tensile tests were performed at 150–250 °C with a strain rate of 0.001 s−1. Tensile creep tests were carried out at temperature of 200 °C and applied stress of 30–50 MPa for Mg–0.5Ce alloys and 50–90 MPa for Mg–2Gd alloys, respectively. Prior to SEM, EDS and EBSD examinations, the sample surfaces were prepared by SiC paper, 6 μm, 3 μm, 1 μm diamond sus- pension and OPS suspension. Te step size used in EBSD tests was 0.5 μm. Data obtained from the EBSD tests were analyzed using TSL OIM sofware. TEM observation was carried out using JEM 2100F TEM at 200 kV and HAADF-STEM mode of JEOL-ARM200F microscope with a probe-forming lens corrector. All TEM samples were water quenched immediately afer creep tests and then prepared by dimple grinding and ion-milling using a Gatan precision ion polishing system (PIPS II MODEL 695). Results and discussion Figure 1 shows the initial microstructure of the as-extruded Mg–0.5Ce and Mg–2Gd alloys. As can be seen, the two alloys exhibit similar average grain size, i.e., 9.7 μm for Mg–0.5Ce and 6.1 μm for Mg–2Gd alloys, respec- tively. (Fig. 1a,d). Te kernel average misorientation (KAM) plot in Fig. 1b,e show that both Mg–0.5Ce and Mg–2Gd alloys have low residual strain. KAM value has a relationship with geometry necessary dislocations (GNDs) and hence can refer to the residual strain21. Figure 1c,f show the SEM and EDS results for Mg–0.5Ce and Mg–2Gd alloys. During extrusion at high temperature, RE-based dynamic precipitation occurred in both alloys since those secondary precipitations are not found in the alloys afer solution treatment (Fig. S1) and are parallel to the ED. Dynamic precipitates formed are most probably Mg 12Ce (Mg–0.5Ce) and Mg 5Gd (Mg–2Gd) as they are the most stable secondary phases observed in previous studies 22,23. Figure 2a,e show the tensile curves of the two alloys at the temperatures ranging from 150 to 250 °C. Smooth fow curves are observed for the Mg–0.5Ce alloys. However, the Mg–2Gd alloys exhibit serrated fow at the temperatures of 150 °C and 200 °C and the serrations disappear at 250 °C. Extensive studies have illustrated that those serrated curves at high temperature are known as PLC efect, caused by the drag force of difusion solute atoms which pin the dislocations temporarily and then raise the stress to reactive them10,11. For the purpose of investigating the interactions between dislocations and solute atoms during creep process, the temperature Scientifc Reports | (2021) 11:2860 | https://doi.org/10.1038/s41598-021-82517-5 2 Vol:.(1234567890) www.nature.com/scientificreports/ Figure 2. Mechanical behaviours of Mg–0.5Ce (the frst row) and Mg–2Gd alloys (the second row) at high temperature: (a,e) tension curves at the temperature ranging from 150 to 250 °C, (b,f) tension creep curves at 200 °C and various applied stress, in which squares mark the point that testing is terminated artifcially, (c,g) creep rate vs. time images, (d,h) log steady state strain rate plotted as a function of log stress. Ratio of the applied stress to the yield stress Minimum creep rate Samples Temperature ( °C) Applied stress (MPa) at 200 °C (%/h) Creep life (h) 30 0.55 0.14 60 Mg–0.5Ce alloys 200 40 0.73 0.51 23 50 0.91 1.20 10 50 0.54 0.0025 > 330 Mg–2Gd alloys 200 70 0.76 0.0091 > 330 90 0.98 0.0144 > 330 Table 1. Summary of the creep properties of Mg–0.5Ce and Mg–2Gd alloys. of 200 °C is chosen to carry out the creep tests since at this temperature serrated fow curve is detected for the Mg–2Gd alloys but not for the Mg–0.5Ce alloys. Additionally, the yield stress for the two alloys at 200 °C is dif- ferent, i.e., 55 MPa for Mg–0.5Ce alloys and 92 MPa for Mg–2Gd alloys.
Details
-
File Typepdf
-
Upload Time-
-
Content LanguagesEnglish
-
Upload UserAnonymous/Not logged-in
-
File Pages8 Page
-
File Size-