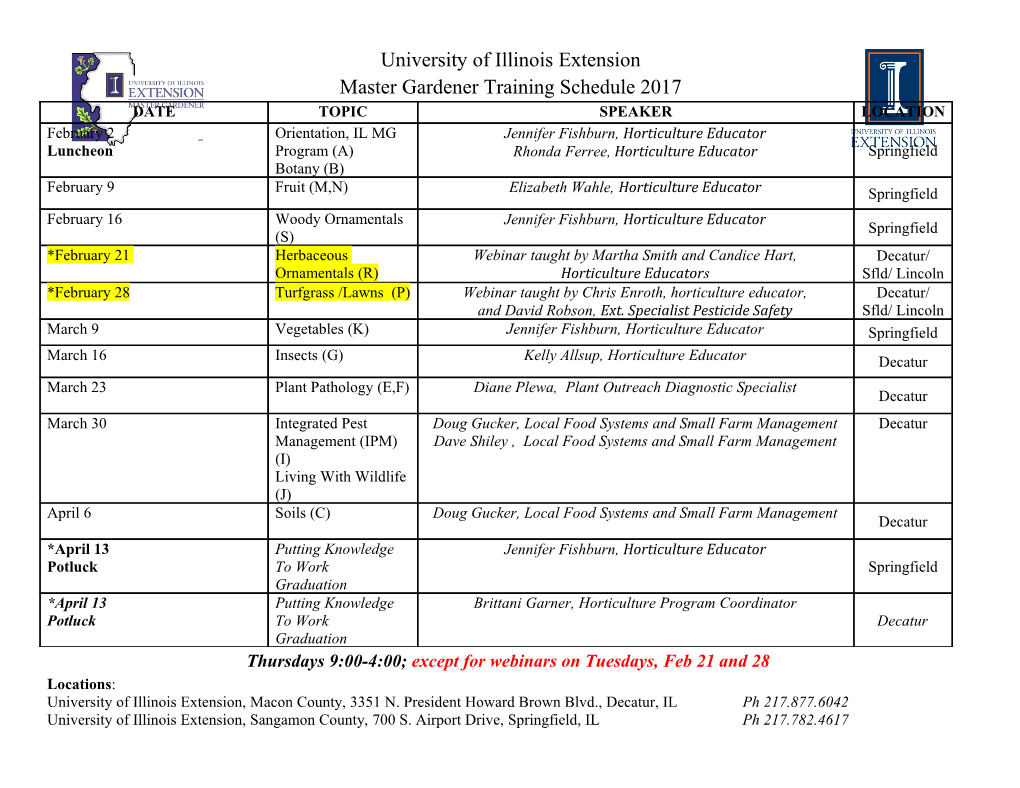
3. Extrasolare Planeten 1 Tagesanzeiger NZZ News vom 22./23. Februar 2017 Wir kennen heute >5000 Exoplanten und Exoplaneten- Kandidaten 3 3.1 Radialgeschwindigkeitsmethode Stern und Planeten kreisen um den Schwerpunkt des Systems. Für die Halbachsen und Massen gilt: MP aP = MS aS Durch RV-Messungen des Sterns kann der Planet nachgewiesen werden. Erste Entdeckung 1995 durch Mayor and Queloz (Obs. Genf): 51 Peg b: P = 4.2 Tage KS = 60 m/s aP sin i = 0.05 AE MP sin i = 0.46 MJ 4 Multiplanetensystem HD40307: 4ME mit P=4.3d, 7ME mit 9.6d, 9ME mit 20.5d Entdeckungen: • “Heisse Jupiters” mit P < 10 d und Teq > 1000 K, • Super-Erden mit M = 3 – 10 ME, • viele Systeme mit mehreren Planeten, • viele Planetenbahnen mit grosser Bahn-Exzentrizität ε > 0.2 5 Beispiel eines Orbits mit grosser Exzentrizität Tamuz et al. 2008, A&A 480, L33 G5 V star P = 527 days K = 97 m/s e = 0.90 MP sin i = 1.56 MJ a sin i = 1.28 Der zusätzliche „Trend“ der RV Kurve verrät einen zusätzlichen Braunen Zwerg / Massearmer Stern mit >20 Jahre Periode! Was haben wir bisher von Radialgeschwindigkeitsprogrammen gelernt? M. Mayor et al.: The HARPS search for southern extra-solar planets Table 1. Occurrence frequency of stars with at least one planet in the defined region. The results for various regions of the m2 sin i log P plane are given. − Mass limits Period limit Planetary rate based on Planetary rate Comments published planets including candidates > 50 M < 10 years 13.9 1.7% 13.9 1.7% Gaseous giant planets > 100 M⊕ < 10 years 9.7 ±1.3% 9.7 ±1.3% Gaseous giant planets > 50 M ⊕ < 11 days 0.89± 0.36 % 0.89 ± 0.36 % Hot gaseous giant planets Any masses⊕ < 10 years 65.2± 6.6% 75.1± 7.4% All ”detectable” planets with P < 10 years Any masses < 100 days 50.6 ± 7.4% 57.1 ± 8.0% At least 1 planet with P < 100 days Any masses < 100 days 68.0 ±11.7% 68.9 ±11.6% F and G stars only Any masses < 100 days 41.1 ± 11.4% 52.7 ± 13.2% K stars only < 30 M < 100 days 47.9± 8.5% 54.1± 9.1% Super-Earths and Neptune-mass planets on tight orbits < 30 M⊕ < 50 days 38.8± 7.1% 45.0 ± 7.8% As defined in Lovis et al. (2009) ⊕ ± ± Table 2. Comparison of detected planets detected and occurrence rate of the ⌘ Earth survey (Howard et al. 2010) and HARPS- CORALIE survey. The comparison is restricted to planets with orbital periods smaller than 50 days. N1 stands for the numbers of detected planets, N2 stands for the number of candidates, and N3 represents the estimated occurrence rate of planets in the given mass range. Mass range HARPS & CORALIE survey ⌘ Earth survey Nb of planets Planetary rate Nb of planets Planetary rate M N1 N2 N3 [%] N1 N2 N3 [%] 3-10⊕ 19 2 48.5 16.6 4.4 5 3 10.2 11.8 4.3 10-30 25 1 20.6 11.1 ± 2.4 4 1 4.6 6.5 ±3.0 30-100 5 1 4.6 1.Mayor17 ±0.52 et al.2 2011 (http://arxiv.org/pdf/1109.2497v1.pdf)1.6 ± 1.2 100-300 4 0 0.8 0.58 ± 0.29 2 1.6 ± 1.2 300-1000 20 0 0.24 ± 0.17 2 1.6 ± 1.2 ± ± 4.3. The mass distribution 100% 95% 1000. 80% 0.2% ± 0.2 60% 40% 0.6% ± 0.3 20% On Fig.10 we have plotted the histogram of masses of the plan- 10% 100.0 ets detected in our sample. We observe a drastic decline of 1.2% ± 0.5 5% the observed mass distribution from about 15 to 30 M . If we 2% limit the range of orbital periods and only consider planets⊕ with 11% ± 2 P < 100 days (Fig. 11), a region where the detection bias are not too important for low-mass planets, we immediately observe the 10.0 17% ± 4 preponderant importance of the sub-population of super-Earths and Neptune-mass planets in that domain of periods. After cor- M2sini [Earth Mass] 24% ± 12 rection of detection biases (Fig. 12), we see even more clearly the importance of the population of low-mass planets on tight orbits, 1.0 with a sharp decrease of the distribution between a few Earth masses and 40 M . We note that the planet population synthe- ⇠ ⊕ 10+0 10+1 10+2 10+3 10+4 sis models by Mordasini et al. (2009b) predicted such a mini- mum in the mass-distribution at precisely this mass range. They Period [days] also pointed out that a radial-velocity measurement precision of 1 about 1 ms− was required in order to detect this minimum. In the Fig. 9. Same as Fig. 6. The dashed lines represent the boxes in framework of the core accretion model, this can be understood which the occurrence rate is computed as defined by Howard by the fact that this mass range corresponds to the runaway gas et al. (2010). An additional box is shown for masses between 1 accretion phase during which planets acquire mass on very short and 3 M . ⊕ timescales. Therefore, unless timing is such that the gaseous disk vanishes at this moment, forming planet transits quickly through this mass range and the probability to detect these types of plan- ets is reduced correspondingly. In Fig. 12 the importance of the (Bonfils & al. 2011). Despite the rather limited range of stel- correction of the detection biases below 20 M is only the re- lar masses in our sample, we have tried a comparison of the flection of the present observing situation for which⊕ only a lim- (m2 sin i log P) distribution for dwarf stars of spectral type F ited fraction of the sample has benefited from the large enough and G versus− the distribution for K dwarfs. The observed di↵er- number of HARPS measurements, required to detect small-mass ence of planetary rate (for periods smaller than 100 days) for the objects. Part of this correction is also related to the growing im- two spectral types is however not significant (Table 1). portance of the sin i e↵ect with decreasing masses. 8 Die “Metallizitaet” der Sterne korrelliert posiv mit dem Vorhandensein von Gasriesen from Fischer and Valenti (2005) [Fe/H] = log [Fe/H]star – log [Fe/H]sun In-class acevity 1 Könnt Ihr abschätzen, wie gross das Radialgeschwindigkeitssignal ist, das Jupiter auf unsere Sonne ausübt? Nehmt an der Orbit ist kreisförmig und man sieht ihn edge-on (also maximales Signal!). Nützliche Info: aJupiter = 5.2 AE MasseJupiter ~ 1/1000 MasseSonne 1 AE ~ 1.5e8 km UmlaufperiodeJupiter ~ 12 Jahre 1 Jahr = 8760 h 9 3.2 Transitmethode HAT-P-7b (Borucki et al. 2009) Bei der Transitmethode werden periodische Sternverdunkelungen durch einen Planeten beobachtet: • Die Methoden eignet sich vorallem für kurzperiodische Planeten, • nur Planeten mit Inklination i ≈ 90o verursachen einen Effekt, 2 • Der relative Effekt ist etwa (RP/RS) , ca. 1% für Jupiter, 0.01% für Erde, • Die Bedeckung des Planeten und die Phase des Planeten kann für ideale Fälle auch beobachtet werden. 10 Die Geometrie von Planetentransit und Planetenbedeckung ! KEPLER: >2000 Planeten um ≈150‘000 Sterne NASA 12 19.10.2016 3. Extra-solare Planeten, H.M. Schmid Beispiel: Der erste Transit-Planet HD 209458 b HD 209458 b erste Transit Messung (von Charbonneau et al. 2000, ApJL 529, L45) Nachfolgebeobachtungen mit Hubble (von Brown et al. 2001, ApJ 552, 699) HAT-P-7 b: Die „Phasen“ eines Exoplaneten (mit Kepler) KEPLER liefert beste Stasek fuer das Vorhandensein von Planeten verschiedener Groesse RJ RN (von Batalha et al. 2013, ApJS 204, 24) KEPLER liefert beste Stasek fuer das Vorhandensein von Planeten verschiedener Groesse Die Dichte von Planeten kann durch Kombinaon von Radialgeschwindigkeit und Transit-Methode besmmt werden. (von Lissauer et al. 2014) Kepler hat bereits einige Planeten idenefiziert, die nah / in der “Habitablen Zone” ihres Sterns kreisen Transmissions-Spektroskopie von “Heissen Jupitern”: Die “Tiefe” eines Transits, bzw. die scheinbare Groesse des Planeten als Funkon der Wellenlaenge, kann Hinweise auf atmosphaerische Zusammensetzung liefern. (von Madhusudhan et al. 2014 Basirend auf Daten des Hubble Teleskops) Die Eigenschaen der Planeten unseres Sonnensystems in Bezug auf die Transit-Methode 3.3 Imaging von Extra-solaren Planeten Ein Abbild eines Planeten gibt wichtige Informationen: • Eigenschaften der Atmosphäre und/oder Oberfläche, • Zusammensetzung, • Variationen durch Rotation, Jahreszeiten, Wetter etc., • Anzeichen von Leben. HR 8799 b,c,d,e (C. Marois et al., 2010) 21 19.10.2016 3. Extra-solare Planeten, H.M. Schmid Spektrale Energieverteilung von Planeten Junge Planeten sind noch in der Kontrakeonsphase. Sie gewinnen dadurch poteneelle Energie und sind deshalb hell. λ 10 Myr log I Thermische Emission 100 Myr Reflektiertes Licht 1 Gyr 0.5 1.0 2.0 4.0 8.0 λ [µm] 2 • reflekeertes Licht ≈ (Rp/dp) Lstar • thermisches Licht ≈ 1/tPlanet (junge Planeten in Kontrakeonsphase) 2 ≈ (Rp/dp) Lstar (alte Planeten) 22 19.10.2016 3. Extra-solare Planeten, H.M. Schmid Die Planeten unseres Sonnensystems gesehen 164 aus 10 pc Enernung DES MARAIS ET AL. FIG. 1. Model spectrum of the sun and planets as seen from a distance comparable to that of a nearby star (10 pc), shown in physical units. Simple Planck emission and wavelength-inde- pendent albedo reflectance components are shown. For Earth, a pure molecular absorption spectrum is superimposed for reference. Des Marais et al. 2002 FIG.
Details
-
File Typepdf
-
Upload Time-
-
Content LanguagesEnglish
-
Upload UserAnonymous/Not logged-in
-
File Pages42 Page
-
File Size-