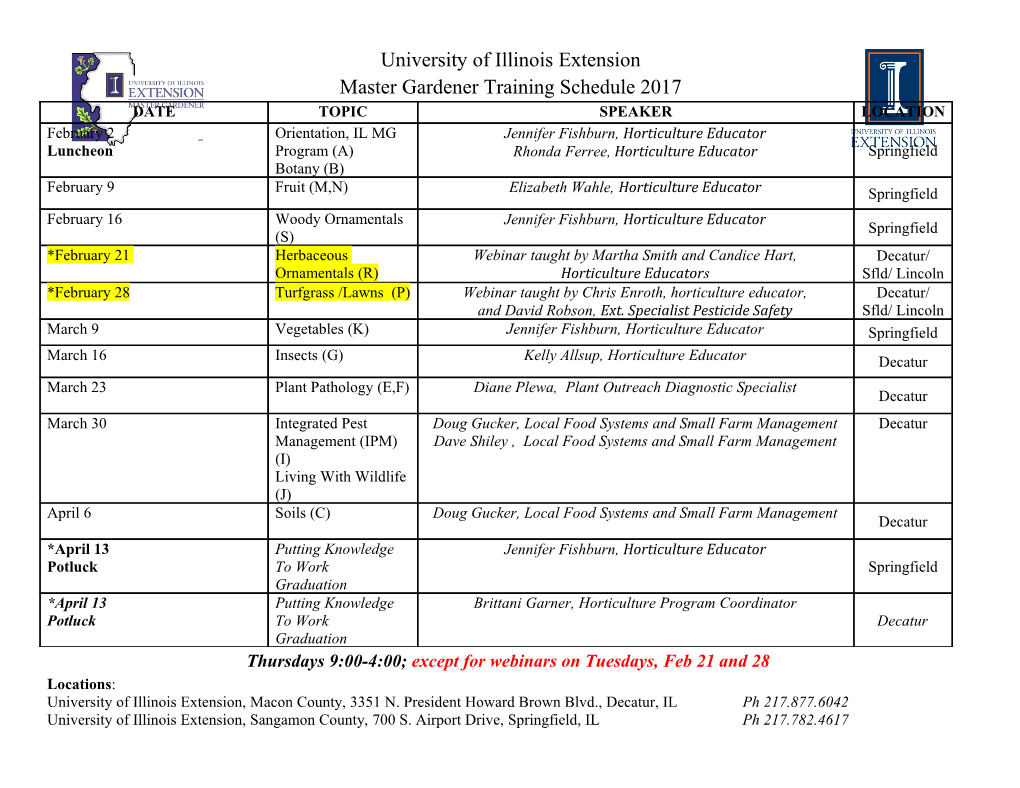
GUIDE TO THE ZARELAB July 2010 Department of Chemistry, Stanford University Welcome to the ZARELAB. This booklet has been prepared to make your visit with us more rewarding by presenting a survey of our recent research activities. Each section was written by those members pursuing the work described therein. Please feel free to ask the members of Dick Zare and the rocket test. my group to discuss any project. INSIDE THIS GUIDE On page 16 is a list of all members of the Zare group as of July 23, 2010 and Table of Contents 2 information on how to contact them. On Research Activities 3 pages 18 through 20 are floor plans of Reaction Dynamics offices and labs in the Mudd Building and Absorption Spectroscopy the Clark Center. The last pages show Mass Spectroscopy Microfluidics maps of the Stanford campus and its Nanoparticles vicinity. Selected Recent Publications 13 Do enjoy your visit! Group Members 16 Floor Plans of Offices and Labs 18 Maps of Stanford Campus & Vicinity 21 TABLE OF CONTENTS Reaction Dynamics 3 State-to-State Reaction Dynamics Nate Bartlett, Justin Jankunas, Tapas Goswami, Nandini Mukherjee Absorption Spectroscopy 4 Measurement of Carbon Isotope Ratios Using Cavity Ring-Down Spectroscopy Doug Kuramoto Mass Spectrometry 5 Two-step Laser Mass Spectrometry of Terrestrial and Extraterrestrial Materials Amy Morrow, Hassan Sabbah 6 Hadamard Transform Time-of-Flight Mass Spectrometry Griffin Barbula, Richard Perry, Konstantin Chingin 7 Unfolding Reaction Pathways in Liquids on the Millisecond Time Scale Using Desorption Electrospray Ionization Richard H. Perry, Konstantin Chingin, Griffin Barbula Microfluidics 8 Single-cell Analysis on a Microfluidic Platform Eric Hall, Peng Guo, Romana Schirhagl 9 Development of a Cell Sorter Based on Integration of Porous Membranes into Layered Microfluidic Devices Romana Schirhagl Nanoparticles 10 Nanoparticle Formation Using Supercritical Fluid Technology Gunilla B. Jacobson, Tatsiana Lobovkina, Jun Ge, Peng Guo, Thomas Cahill 11 Encapsulation of Water-Soluble Compounds in Polymer Nanoparticles by Supercritical Carbon Dioxide Antisolvent Precipitation Jun Ge, Gunilla B. Jacobson, Tatsiana Lobovkina 12 Lipid Nanoparticles for Sustained Drug Delivery Tatsiana Lobovkina 13 Nanopore-Injection Method for Generating Organic Nanoparticles Peng Guo Selected Publications 14 Zare Group Contact List 16 Office/Lab Floor Plans 18 Maps of Stanford & Vicinity 21 2 STATE-TO-STATE REACTION DYNAMICS Nate Bartlett, Justinas Jankunas, Tapas Goswami, Nandini Mukherjee Using a home-built 3D ion imaging experimental apparatus1 we were previously able to study reactive and inelastic scattering in an H + D2 reaction to give HD (v′=1,3, j′) or D2 (v′=1-4, j′), respectively. The products of interest were state-selectively ionized using [2+1] resonance-enhanced multiphoton ionization (REMPI). The resulting ions were accelerated toward a multichannel plate (MCP) coupled to a delay-line anode which measures the 3D velocity distribution of the reaction products which can then be converted to a differential cross section (DCS) using the PhotoLOC (photoinitiated reaction analyzed with the law of cosines) technique developed in the Zarelab. The experimental results on reactive scattering in an H + D2 → HD (v′=1, j′) + D reaction agreed well with the theoretical predictions.2 As the rotational excitation of HD increased the DCS shifted from backward to sideward scattering, again in an agreement with the theoretical predictions. H + D2 → HD (v′=3, j′) + D reaction exhibited an even richer dynamics.3 In particular, time-delayed forward scattering was attributed to a glory effect that resulted from a near- and far-side quantum interference. Inelastic scattering experiments also revealed a few interesting features.4 It was shown, for example, that the translational H atom energy was transformed into a vibrational D2 energy mainly via a bond elongation with forward scattered products rather than bond compression and backward scattered products. Additionally, it is expected that the spatial distribution of the rotational angular momentum J of collision products is not isotropic. In other words the M-states of the reaction products are expected to be populated unequally. We are actively working towards the goal of measuring DCS and product state distributions M-state selectively. By selecting the initial and final states of the REMPI process, as well as the polarization of light used, one can detect molecules M-state selectively. This technique has been successfully demonstrated recently 5,7 for pure samples of H2, HD, and D2 in our lab. However, it turns out that the weak line strengths of the required REMPI lines and the relatively small reaction cross section of the H + D2 reaction make this technique extremely difficult to apply to reaction products. There is another way to detect molecules M-selectively which we are currently exploring. In the presence of a strong electric field, the rotational levels of molecules are shifted and split into |M|-resolved components due to the Stark effect. Commercial Nd:YAG lasers can readily produce the fields required to produce this splitting. By syncing a “Stark” laser pulse with our product ionization laser we hope to obtain the first M - resolved DCS for the H + D2 reaction. Our section is also constantly exploring other interesting and exciting paths. For example we recently finished recording product branching ratios for the D + DBr → D2 + Br/Br* reaction, where Br* represents spin-orbit excited bromine.6 We found that as expected, there is negligible product formation through the non- adiabatic reaction pathways. We are also excited to return to working on polyatomic systems with the H + neopentane (C(CH3)4) reaction. We encourage you to read more about these exciting experiments below! References: 1. K. Koszinowski, N. T. Goldberg, A. E. Pomerantz, and R. N. Zare, J. Chem. Phys. 125, 133503 (2006) 2. K. Koszinowski, N. T. Goldberg, J. Zhang, R. N. Zare, F. Bouakline, and S. Althorpe, J. Chem. Phys. 127, 124315 (2007) 3. N. T. Goldberg, J. Zhang, D. J. Miller, and R. N. Zare, J. Phys. Chem. A 112, 9366 (2008) 4. N. T. Goldberg, J. Zhang, K. Koszinowski, F. Bouakline, S. Althorpe, and R. N. Zare, PNAS 105, 18194 (2008) 5. N. C. M. Bartlett, D. J. Miller, R. N. Zare, D. Sofikitis, T. P. Rakitzis, and A. J. Alexander, J. Chem. Phys. 129, 084312 (2008) 6. J. Zhang, J. Jankunas, N. C.-M. Bartlett, N. T. Goldberg, and R. N. Zare, J. Chem. Phys. 132, 084301 (2010) 7. N. C.-M. Bartlett, J. Jankunas, R. N. Zare, and J. A. Harrison, Phys. Chem. Chem. Phys. (in press, 2010). 3 MEASUREMENT OF CARBON ISOTOPE RATIOS USING CAVITY RING-DOWN SPECTROSCOPY Doug Kuramoto The measurement of isotopes ratios, such as 13C/12C, is important in chemistry and other fields, such as geology, since it provides useful information on formation and transport processes. The most common method used to measure isotope ratios is the isotope ratio mass spectrometer (IRMS), which is bulky, requires user expertise, and is costly. The measurement of isotope ratios can also be done through absorption spectroscopy using cavity ring-down spectroscopy (CRDS).1 We have developed an instrument capable of measuring carbon isotope ratios in organic samples (Fig. 1A). The sample is injected into a gas chromatograph (GC) where it is separated into its components. The effluent is passed through a catalytic combustor (C) consisting of platinum and oxidized nickel wires in a ceramic tube heated to 1150 ºC, which completely oxidizes the carbon in the sample to carbon dioxide. The combustion products are fed into a CRDS instrument to measure the concentrations of 12C16O16O and 13C16O16O. We refer to our setup as GC-C-CRDS. A mixture of methane, ethane, and propane injected in our instrument can be separated, oxidized, and measured as shown in Fig. 1B. The chromatographic peaks are used to determine the ratio of 13C/12C in the sample. The current instrument can measure isotope ratios with a precision of less than one part per thousand and an accuracy of less than a few parts per thousand. Our instrument does not currently match the capabilities of instruments based on IRMS, but we expect future improvements to make our instrument an attractive alternative to IRMS. Despite the present limitations, the performance is sufficient for certain applications such as those in the oil industry. We are currently measuring isotope ratios of hydrocarbon gasses produced from petroleum sources which can provide useful information for the characterization of an oil reservoir. Figure 1. (A) The GC-C-CRDS instrument. The sample is separated using gas chromatography and combusted to produce carbon dioxide and then the isotopic carbon dioxide concentrations are measured using cavity ring-down spectroscopy. (B) Chromatograms of 12C16O16O and 13C16O16O produced from a mixture of methane, ethane, and propane. References: 1 R. N. Zare, D. S. Kuramoto, C. Haase, S. M. Tan, E. R. Crosson, and N. M. R. Saad, Proc. Natl. Acad. Sci. (US) 106, 10928-10932 (2009). 4 TWO-STEP LASER MASS SPECTROMETRY OF TERRESTRIAL AND EXTRATERRESTRIAL MATERIALS Amy Morrow, Hassan Sabbah Microprobe laser-desorption laser-ionization mass spectrometry (μL2MS) is a powerful and versatile microanalytical technique that is used to study organic molecules in situ in a wide range of terrestrial and extraterrestrial materials. The combination of focused laser-assisted thermal desorption and ultrasensitive laser ionization provides sensitivity, selectivity, and spatial resolution capabilities that are unmatched by traditional methods of analysis. Over the past decade, this laboratory has developed and applied the μL2MS technique in a number of different research projects. Some areas that we are currently focusing on are: Instrument Development: To enhance the analytical ability of the μL2MS technique we are actively pursuing instrument developments. Our plans include: the addition of a camera to visualize desorption and development of the neutral plume; installation of a single photon ionization source to allow detection of PAHs without dependence on resonant absorption; and application of soft ion landing techniques to attempt to separate complex mixtures of species by mass.
Details
-
File Typepdf
-
Upload Time-
-
Content LanguagesEnglish
-
Upload UserAnonymous/Not logged-in
-
File Pages23 Page
-
File Size-