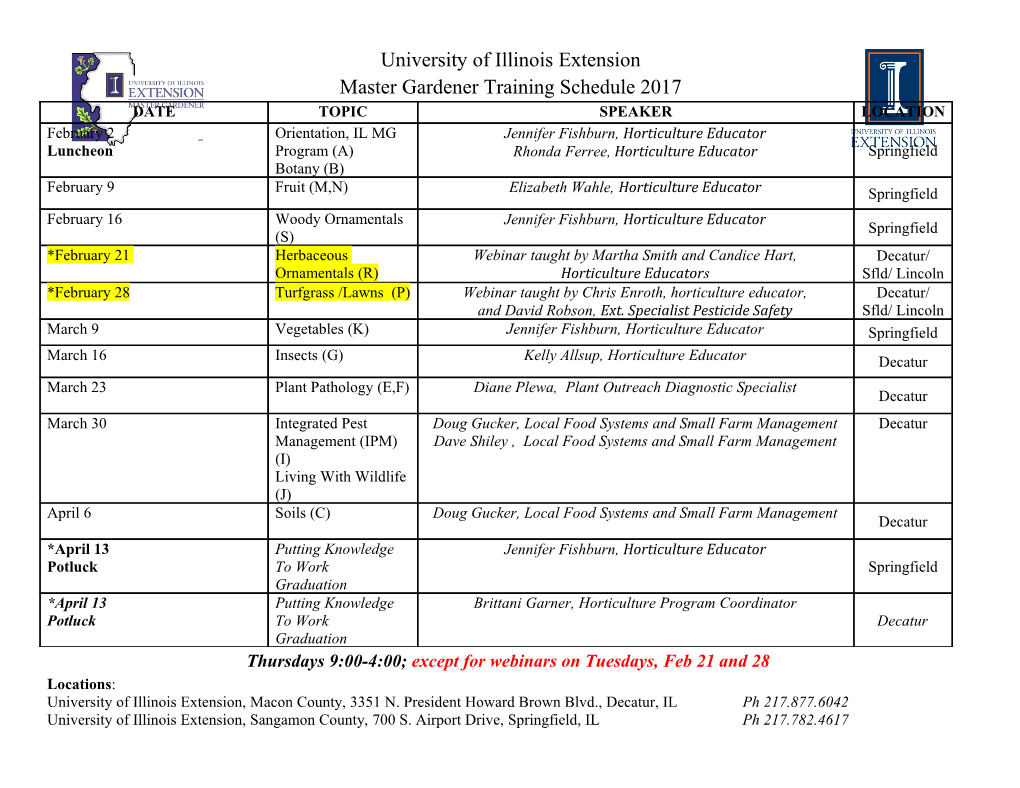
catalysts Article Synthesis and Characterization of N-Doped SiC Powder with Enhanced Photocatalytic and Photoelectrochemical Performance Wanli Liu, Qi Li, Xianglong Yang *, Xiufang Chen and Xiangang Xu State Key Laboratory of Crystal Materials, Shandong University, Jinan 250100, China; [email protected] (W.L.); [email protected] (Q.L.); [email protected] (X.C.); [email protected] (X.X.) * Correspondence: [email protected]; Tel.: +86-151-6505-7949 Received: 2 June 2020; Accepted: 6 July 2020; Published: 9 July 2020 Abstract: Solar-light-driven N-doped 3C–SiC powder was synthesized via a simple one-step combustion route. SiC–N photocatalysts exhibited 205.3 µL/(g h) hydrogen evolution rate, nearly 2 · 2 times that of SiC–Ar(120.1 µL/(g h)), and was much higher than that of SiC nanowires (83.9 µL/(g h)), · · SiC nanoparticles (82.8 µL/(g h)) as well as the B-doped SiC photocatalysts(166 µL/(g h)). In cyclic · · tests, N-doped SiC also performed excellent photocatalytic durability and good structural stability. It can be concluded that the influence of N-doping introduced defects into the SiC photocatalyst by occupation and mixed phase structure, transformed the band structure into the direct band gap, and formed a shallow donor level for trapping holes. Consequently, higher photocatalytic activities and lower recombination was achieved. Furthermore, the carbon on the photocatalyst which was yielded from the substitution of N or which remained after combustion would build constructed efficient interfacial contact with SiC for the quickening of light-driven electron transfer to the surface, and simultaneously strengthen the adsorption capacity and light-harvesting potential. Keywords: N-doped; SiC; photocatalysis; hydrogen production 1. Introduction Confronted with the dual pressures of a resource crisis and environmental pollution, the sustainable development and use of clean energy has become a hot issue [1]. The conversion of solar energy into hydrogen via water-splitting using a semiconductor as a photocatalyst has been considered to be an ideal and promising processes for the generation of clean and renewable energy [2,3]. To date, varieties of materials for photocatalytic application have been widely studied since Fujishima and Honda initially reported photoelectrochemical hydrogen production using TiO2 [4]. Similar to TiO2, many other metal oxides are also active for water-splitting, but a wide band gap and low quantum efficiency has a negative impact with regard to the efficient use of sunlight [5]. Non-oxides possess the band gap necessary to absorb visible light, but are generally unstable and prone to photo-corrosion and self-oxidation [6]. Therefore, it is still urgently necessary to develop photocatalysts that can use visible light and that are highly stable, as well as highly efficient and less expensive [7]. Silicon carbide (SiC) had an appropriate and tunable band gap (2.4–3.2 eV) [8] and a relatively negative conduction band position for visible-light-driven photocatalytic hydrogen evolution. Moreover, it exhibits high charge-carrier mobility, excellent thermal and chemical stability, and is environmentally friendly and abundant in low-cost raw materials [9]. However, SiC does not exhibit a satisfying photocatalytic action due to its low hydrogen production efficiency caused by an indirect + band gap, lack of active sites, and high recombination rate of photo-excited e−/h pairs [10,11]. Catalysts 2020, 10, 769; doi:10.3390/catal10070769 www.mdpi.com/journal/catalysts Catalysts 2020, 10, 769 2 of 12 To address these challenges and improve the photocatalytic performance of SiC catalysts, various engineering strategies, such as morphology or structure control [12–14], non-metal doping [15,16], noble metal deposition (Au, Ag, Pt, etc.) [17–19], construction of combination and heterostructures (e.g., TiO2/SiC [20], SnO2/SiC [21], MoS2/SiC [22], SiC/CdS [23–25], g-C3N4/SiC [26], and SiC/BiVO4 [27], and hybridization of carbon materials (e.g., carbon nanotubes-SiC, graphene-SiC hybrids, and graphitic carbon-SiC [28–31] have been manufactured. However, the preparation of SiC photocatalyst that is efficient, stable, and visible-light-driven via a simple method remains a challenge. Ion-doping has proved to be a valid and widely used way to improve photocatalytic activity by expanding the + light-response range and altering the recombination rate of photo-excited e−/h pairs. Nitrogen is widely used as an n-type dopant in silicon carbide due to its similarity in atomic radius compared to a C atom. For N-doped SiC, studies have mainly focused on the diffusion of N in SiC [32] and the dielectric and optical properties of N-doped SiC [33]; the effect of N-doping in SiC for photocatalytic H2 production under visible light has not been reported so far. Along with gradual occupation by N atoms at the C site, Si3N4 is also produced as an important intermediate product during the N-doping process. As the crystallization of SiC is hindered by the formation of Si3N4, the grain size is lessened, which improves chemical activities. At the same time, the reduction of Si3N4 raises the N pressure around the SiC lattice, which urges the occupation of N to form SiCN solid solution and yields graphite C, which enriches the diversity of the phases. The band structure transforms into the direct band gap with band-gap shrinkage due to the capture of unmatched electrons by Si 3p after N substitution. Additionally, separation photoelectron holes, promoted by heterostructure and interfacial contact, form in the photocatalyst. Herein, a simple combustion synthesis process combining ion-doping and carbon-coating was adopted to prepare N-doped SiC. The as-prepared N-doped 3C-SiC photocatalyst was obtained with an enhanced activity as high as 205.3 µL/(g h) for H production under solar light, which is nearly · 2 2 times that of SiC–Ar (120.1 µL/(g h)), and was much higher than that of SiC nanowires (83.9 µL/(g h)), · · SiC nanoparticles (82.8 µL/(g h)), and the B-doped SiC photocatalysts (166 µL/(g h)). The chemical · · state, morphology, phase structure, optical properties, and the band structure of N-doped SiC were also investigated to evaluate the impact of N-doping on the photocatalytic activities. The strategy in this work provides a widely used reference for SiC-based systems to improve related photocatalysis. 2. Results and Discussion 2.1. Synthesis and Characterization The X-ray diffraction (XRD) patterns of the as-prepared samples are shown in Figure1. The characteristic diffraction peaks at 2θ = 35.8◦, 41.3◦, and 60◦ which are indexed as the (111), (200), and (220) facets of cubic SiC (JCPDS, No. 29–1129) appear in both samples, which implies that the main phase structure of SiC does not change significantly after N-doping. For the N-doped sample, the intensities of the peaks mentioned above decrease; additionally, the characteristic peaks of graphite C and Si3N4 weakly appear, indicating that the traces of residual carbon and Si3N4 appear in the product. This is accompanied by the direct formation (1) of SiC: Si (l, s) + C (s) = SiC (s) (1) Si3N4 also came out as an intermediate product as shown in reaction (2), 3Si (s) + 2N2(g) = Si3N4 (s) (2) which not only retarded the generation of SiC owing to its more thermodynamic activity under 1550 ◦C[34], but also resulted in higher local N pressure in the SiC lattice, which accelerated the occupation by N atoms on C atoms and produced a SiC(N) solid solution. Consequently, the excess C, which is replaced by N, yielded graphite C in the final product. When the carbon was sufficient under Catalysts 2020, 10, 769 3 of 12 Catalysts 2020, 10, x FOR PEER REVIEW 3 of 13 an inert atmosphere and above the boundary temperature 1450 ◦C, the conversion from Si3N4 to SiC would be continuous until complete (3) [35]. atmosphere and above the boundary temperature 1450 °C, the conversion from Si3N4 to SiC would be continuous until complete (3) [35]. Si3N4 (s) + 3C = 3SiC (s) + 2N2 (g) (3) Si3N4 (s) + 3C = 3SiC (s) +2N2 (g) (3) The existence of peaks assigned to graphite C and Si3N4 is evidence of the unfinished process. The existence of peaks assigned to graphite C and Si3N4 is evidence of the unfinished process. The detailed enlargement of the peak at 41 corresponding to (200) plane displays a shift towards The detailed enlargement of the peak at 41°◦ corresponding to (200) plane displays a shift towards the higher 2θ angle in the SiC–N2 sample, compared to SiC–Ar, suggesting a narrowed interplanar the higher 2θ angle in the SiC–N2 sample, compared to SiC–Ar, suggesting a narrowed interplanar distance d, and a consequently reduced lattice constant a. Calculated by Jade 5.0 software, the lattice distance d, and a consequently reduced lattice constant a. Calculated by Jade 5.0 software, the lattice constant corresponding to 3C–SiC of SiC–N2 is 4.20 Å, whose value is much smaller than the standard constant corresponding to 3C–SiC of SiC–N2 is 4.20 Å, whose value is much smaller than the standard value 4.36 Å of 3C–SiC, which is consistent with the result of the peak shift. The reason for the value 4.36 Å of 3C–SiC, which is consistent with the result of the peak shift. The reason for the above above change can be summarized as follows: (1) owing to its priority formation below the boundary change can be summarized as follows: (1) owing to its priority formation below the boundary temperature and drop in the combustion temperature when decomposed, Si3N4 restricts the generation temperature and drop in the combustion temperature
Details
-
File Typepdf
-
Upload Time-
-
Content LanguagesEnglish
-
Upload UserAnonymous/Not logged-in
-
File Pages12 Page
-
File Size-