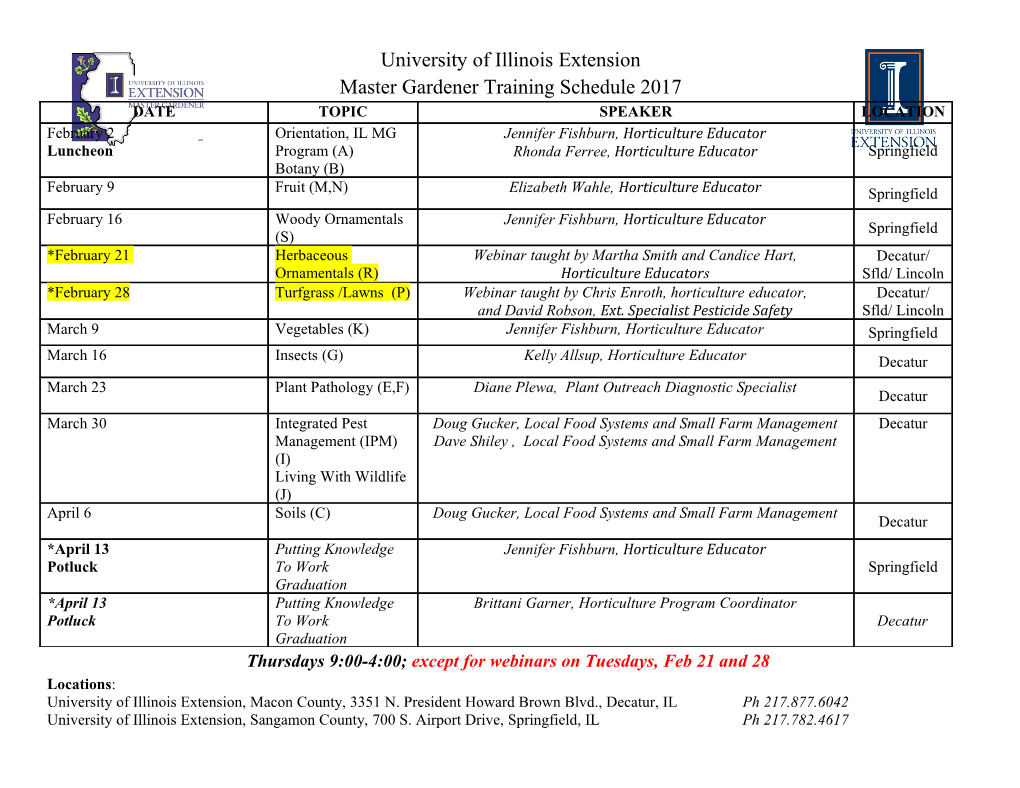
UNIVERSITY OF OKLAHOMA GRADUATE COLLEGE DESIGN AND ANALYSIS OF COMPLIANT WHEELS FOR A PLANETARY ROVER A THESIS SUBMITTED TO THE GRADUATE FACULTY in partial fulfillment of the requirements for the Degree of MASTER OF SCIENCE By BRANDON MILLS Norman, Oklahoma 2007 c Copyright by BRANDON MILLS 2007 ! All Rights Reserved. Acknowledgements I would like to my advisor, Dr. Miller, for his patience, insight, and guidance in the completion of this research. I also offer sincere appreciation to my other committee members, Dr. Baldwin and Dr. Chang. My thanks go to these three, not only for agreeing to serve on my committee, but for all that I learned in their classes as a student. I would also like to give thanks to the rest of the faculty and staff within the School of Aerospace and Mechanical Engineering. I could not have completed my projects and my classwork without these individuals. Thanks especially to machine shop director Billy Mays. I also thank my colleagues, Dan, Tim, and Matt, for their continual encourage- ment and advice. Sincere appreciation goes to Matt, who was always available to give assistance, and without whose help I would still be in the machine shop trying to learn how to use the machines. I have to thank my friends for ensuring that I didn’t miss out on “the college experience.” Finally, I would like to thank my parents, and the rest of my family, without whose love and endless support I would not be here today. iv Contents List Of Tables vii List Of Figures viii 1 Introduction 1 1.1 Existing Rover and Wheel Designs . 1 1.1.1 Rovers on Other Planets . 1 1.1.2 Rover Prototypes . 11 1.2 Compliance . 18 1.3 Design Goals . 18 1.4 Problem Statement and Organization of Thesis . 20 2 Design Constraints 22 2.1 Fall Height . 22 2.2 Spaceworthiness . 24 2.3 Rolling Efficiency . 27 3 Flexure Wheel 31 3.1 Design . 31 3.2 Analysis . 33 3.3 Design Variations . 34 4 Tweel 37 4.1 Spring Rate . 38 4.2 Impact Force . 40 5 Tension-Spring Wheel 42 5.1 Design Considerations . 43 5.2 Computer Terrain Simulation . 50 5.3 Spun Wheel . 53 5.4 Metal Spinning . 55 5.5 Spun Wheel Design . 58 5.6 Wheel Fabrication . 60 v 6 Results and Conclusions 65 6.1 Flexure Wheel Results . 65 6.2 Accelerometer Testing . 67 6.3 Tweel Field Testing . 72 6.4 Tension-Spring Wheel Testing . 75 6.5 Tweel Design Changes . 79 6.6 Tension-Spring Wheel Design Changes . 81 6.7 Conclusions . 84 Reference List 86 Appendix A 90 Data Calculations . 90 Appendix B 91 Mechanical Drawings . 91 vi List Of Tables 2.1 Rolling resistance for various materials . 29 3.1 Flexure dimensions and mass for five wheel designs . 35 3.2 Forces, stresses, and displacements for falling flexure wheel . 36 5.1 Spring specifications and power losses . 48 5.2 Selected alloys and their elongations . 56 6.1 Voltages, currents, and power used by rigid wheel and spring wheel . 78 6.2 Theoretical and actual mass of tension-spring wheel components . 83 6.3 Pro/con table for Tweel and tension-spring wheel . 85 6.4 Comparison of three wheel types . 86 vii List Of Figures 1.1 Lunokhod . 3 1.2 Detail of Lunokhod’s wheels . 3 1.3 Lunar Roving Vehicle . 5 1.4 Detail of Lunar Roving Vehicle wheel . 5 1.5 Sojourner rover flown in Mars Pathfinder mission . 7 1.6 Sojourner wheels, prototype and final design . 9 1.7 Artist rendering of MER rover . 10 1.8 MER wheels . 11 1.9 Early wheel designs for University of Oklahoma rovers . 13 1.10 Carbon fiber wheel mounted on SR-II . 15 1.11 Carbon fiber wheel delaminating in extreme heat . 16 1.12 High-efficiency wheel . 17 2.1 Free-body diagram of wheel rolling across surface . 29 3.1 Five-spoke flexure wheel . 32 3.2 Von Mises stress in five-spoke flexure wheel under 650 N load . 34 3.3 Von Mises stress in eight-spoke flexure wheel under 800 N load . 36 4.1 Michelin Tweel . 37 4.2 Tweel in materials testing machine . 40 4.3 Force vs. displacement for Tweel . 40 5.1 Cross-section of wheel with all springs in one plane . 44 5.2 Cross-section and free-body diagram of wheel . 44 5.3 Testing to find rolling friction of simple wheel model . 48 5.4 Simulated wheel rotation driving across terrain . 52 5.5 Original design for tension-spring wheel . 53 5.6 Diagram of lathe setup for spinning . 57 5.7 Spun wheel, exploded view . 58 5.8 Maximum deflection of tire section under loading . 59 5.9 Spinning setup used for first wheel . 61 5.10 Product of second attempt at spinning . 62 5.11 Rippled wheels from unsuccessful attempts at spinning . 64 viii 6.1 Acceleration during impact from a 5-cm fall with rigid wheel . 68 6.2 Acceleration during impact from a 5-cm fall with Tweels . 70 6.3 Acceleration during impact from a 5-cm fall with tension-spring wheels 71 6.4 Computer model of tweel and hub assembly, exploded view . 73 6.5 Field test of SR-II with Tweels . 74 6.6 Completed tension-spring wheel . 75 6.7 SR-II with tension-spring wheels . 76 ix Abstract Robotic rovers are the foundation for the exploration of planetary bodies. These rovers must be designed to endure long durations in harsh environments. The wheels are a key system on planetary rovers. A rover wheel must meet many requirements: it must be compliant, in order to reduce the stress on the rover’s drivetrain components; it should be made of spaceworthy materials; it needs to provide high traction to traverse soil, sand, and rock; it must be robust; the wheel should have as little rolling friction as possible; and its mass should be minimal. This thesis describes the design and analysis of non-pneumatic prototype wheels for a Mars rover, Solar Rover II (SR-II). Two possible final designs are presented: a Tweel-style design, and a tension-spring wheel design. The Tweel uses thin rubber spokes to absorb energy, and increases the allowable fall height of the rover from 10 cm to 15 cm. The spring wheel design employs an arrangement tension springs to absorb impact forces. When mounted on SR-II, this design allows the rover to safely survive a fall from 18 cm. The total mass of the wheel is 657 g. The increase in x power consumption for the rover is only about 1.5 W for various conditions, including straight-line traverses and skid-steering maneuvers. xi Chapter 1 Introduction Rovers are used to explore harsh space environments where it is too dangerous or too costly to send humans. Unmanned landers can be sent to very precise locations, but most missions can benefit collecting data from multiple sites. The additional freedom and mobility of a rover can be a great advantage. 1.1 Existing Rover and Wheel Designs This section will highlight select rover designs and their mission accomplishments. Technical specifications for the rovers will be presented, and any relevant informa- tion regarding mobility system, specifically, the rovers’ wheel designs, will also be introduced. 1.1.1 Rovers on Other Planets The first rover to traverse an extraterrestrial body was Lunokhod 1. It landed on the moon on November 17, 1970, carried by the Luna 17 spacecraft. Built by the Soviet Union, the rover consisted of a sealed tub-like body and eight, independently- powered wheels. The rover stood 1.35 m high, had a length of 2.30 m, a wheelbase of 1 1.70 m, and a track of 1.60 m. Its mass was a hefty 756 kg. Lunokhod 1 operated on the Moon for almost 11 lunar days1, in which it traveled 10.54 km, transmitted more than 20,000 television pictures, recorded over 200 panoramic photographs, and con- ducted more than 500 soil mechanics tests. The rover was equipped with cone-shaped antenna, a highly-directional helical antenna, one standard television camera, three panoramic television cameras, an x-ray spectrometer, an x-ray telescope, cosmic-ray detectors, and a soil testing apparatus. The rover was teleoperated by a team of five drivers [20, 23]. Lunokhod 2 followed in 1973, on the Luna 21 mission. This rover weighed 840 kg, 84 kg more than its predecessor. Lunokhod 2 was very similar to Lunokhod 1, and covered 37 km in four lunar days. It also took 86 panoramic images and 80,000 television images, and conducted mechanical tests of the lunar soil as Lunokhod 1 did. The main tub-shaped compartment of the Lunokhod rovers was sealed with a convex lid, and pressurized with nitrogen to one atmosphere. The internal tem- perature of the nitrogen was kept between 0o and 40oC by the radioactive isotope Polonium-210. All electronics were housed in this body, and this design allowed 1A lunar day is about equal to one month on Earth, or 29.5 Earth days 2 Figure 1.1: Lunokhod (image reproduced from NASA) Soviet engineers to avoid having to design electronics which could withstand the extreme temperatures and low pressure of space. Solar arrays charged Lunokhod’s batteries during the lunar day, and the rovers would hibernate during the long, cold nights [17]. Figure 1.2: Detail of Lunokhod’s wheels (image reproduced from [17]) Each of the Lunokhod rovers’ wheels had its own suspension, motor, and brake, and any wheel could be disconnected from its motor in the case of a motor failure.
Details
-
File Typepdf
-
Upload Time-
-
Content LanguagesEnglish
-
Upload UserAnonymous/Not logged-in
-
File Pages103 Page
-
File Size-