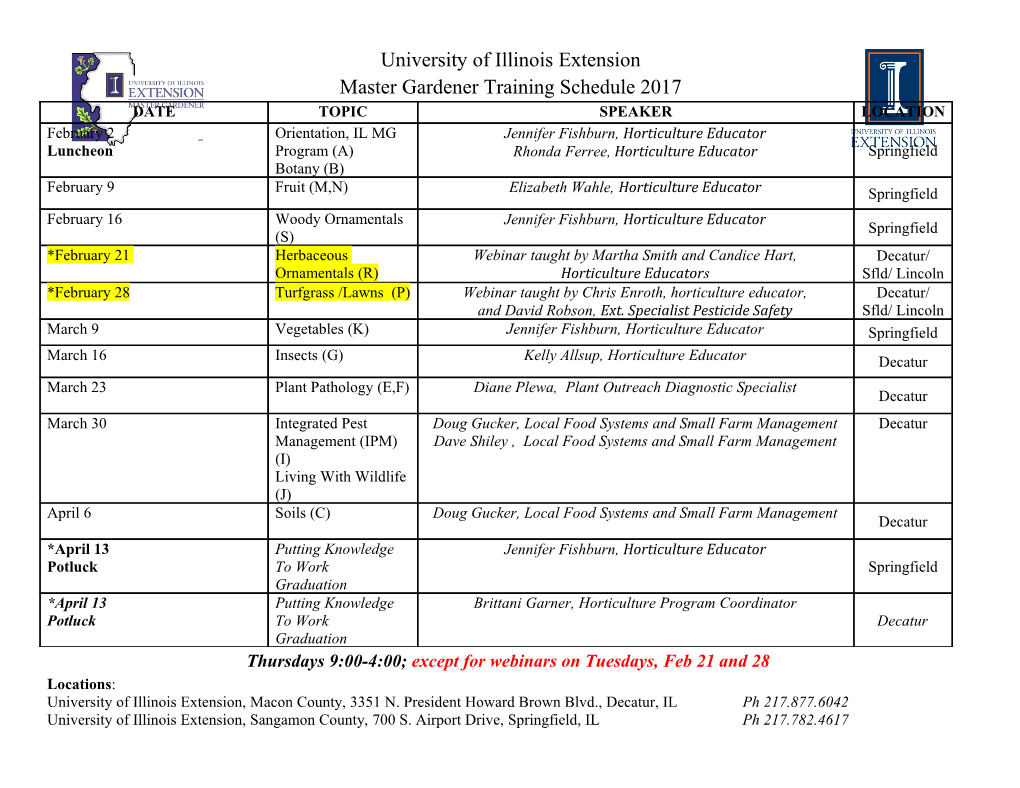
Ninth International Conference on Mars 2019 (LPI Contrib. No. 2089) 6211.pdf POSSIBLE PINGOS AND “WET-BASED” PERIGLACIATION AT THE MARTIAN DICHOTOMY. R.J. Soare1, S.J. Conway2 and J-P Williams3, 1Dept. of Geography, Dawson College, Montreal, Qc, Canada H4B 1Z2 ([email protected]). 2CNRS UMR 6112, LPG, Nantes, France. 3Dept. of Earth, Planetary and Space Sci- ences, University of California, Los Angeles, CA 90095, USA. Introduction: Mounds that exhibit similarities of Observations: In the main, the pingo-like mounds shape, scale, features, and landscape with terrestrial are circular/sub-circular to elongate (Fig. 3). Some pingos (perennially ice-cored mounds) [e.g. 1] (Fig. 1) mounds, however, exhibit irregularities of shape, either have been observed at the mid-latitudes of Utopia Plan- topographically or geometrically. Mound long-axes itia [3-4,6-7,9] and the Argyre region [8], as well as at comprise tens to hundreds of metres. Some mounds the near-equatorial latitude of Athabasca Vallis [2,5]. show summit fractures, that may or may not extend to Pingo formation on Earth largely follows one of the mound bases, and summit depressions. Some two pathways: a) the precursive pooling of surface or mounds are polygonised. Typically, the adjacent terrain near-surface water, its freeze-thaw cycling and perma- is polygonised, dissected or both. Often the frost aggradation (hydrostatic/closed-system pingos polygonised terrain is characterized by shallow wave- [CSPs]); or, b) groundwater migration driven or facili- like incisions. Occasionally, circular to sub-circular tated by topography, geological faulting or artesian depressions absent of rims are observed amidst the pressure (hydraulic/open-system pingo [OSPs]) [1]. waves. 300 m 300 m Fig. 1. OSP/thermokarst-lake landscape in the Tuktoyaktuk Fig. 3. Cluster of possible pingos in localised depression, eastern Coastlands, Beaufort seacoast, Canada (aerial photo A27917- rim of Moreux crater. Note: a) the similarity of shape and sum- 35-1993). Note: the summit depressions and cracks on the two mit fracturing of the largest mound (bottom right-hand corner), largest pingos, Split (centre-bottom) and Ibyuk (right). North is comparable to that observed at Ibyuk and Split pingos (see Fig. to the left. Image credit: National Air Photo Library, Ottawa, 1) and; b) the terrain dissection (upper left-hand side of the im- Canada. age. HiRISE image ESP 058140_2255; 42.1440 N; 45.9640 W. North is up. Image credit: NASA/JPL/ Univ. of Arizona. Here, we report and discuss the occurrence of pin- go-like mounds and possible “wet” -based entourages All of the mounds are located in or immediately ad- of periglacial landforms at the Martian dichotomy jacent to topographical lows (i.e. basins, valleys or within and adjacent to the Moreux crater (42.1o N; channels). The basins are filled in, at least partially, by 44.4o E) (Fig. 2). relatively smooth material (at HiRISE magnification) that blankets and mutes the underlying terrain [13]. Earth analogues: a) Typically, CSPs are observed in areas of continuous, deep and “ice-rich” permafrost where the landscapes are dotted with thermokarst lakes and alases, i.e. thermokarst-lake basins absent of water. The mounds originate with the loss of lake water, by evaporation or drainage, and the exposure of the lake basin to freezing temperatures. As the freezing front propagates downwards through the floor and sideways Fig. 2. MOLA map showing Moreux crater (bottom right). Col- from the margins towards the basin centre, trapped ours indicate elevation, i.e. lighter colour = higher elevation. Image credit: Goddard Space Flight Center, NASA. near-surface pore-water uplifts the sedimentary over- burden (i.e. the newly-exposed lake floor) and a mound A pingo-like origin for the Martian mounds would begins to form. Radial-dilation cracks may propagate be consistent with the growing body of literature point- from the summit as the mound grows, an ice core ing to the possible work of periglaciation in revising forms, and tensile stresses within the overburden in- relatively Late Amazonian Epoch landscapes on Mars crease. Sometimes, these cracks grade into the sur- [e.g. 10-12]. rounding polygonised terrain [1]. Ninth International Conference on Mars 2019 (LPI Contrib. No. 2089) 6211.pdf Pingo heights range from nascent to decametres and Discussion: The terrain surrounding the Moreux long-axis diameters may comprise hundreds of metres. crater, straddling the Mars dichotomy, is complex and Pingo shapes encompass a broad spectrum from circu- fretted; it comprises a jumbled admixture of cliffs; me- lar/sub-circular to elongate or irregular. sas; buttes; straight-walled and sinuous canyons; ubiq- b) Often, OSPs form in areas of marked relief, i.e. uitous lobate-debris aprons; possible glacial-like flow- glacial valleys, and where the local hydrological- features and moraines; and, terrain seemingly desic- system is active, and within the foregrounds or outwash cated of icy material by sublimation at some locations plains of ice-sheets, glaciers or caps [e.g. 1,14]. OSP yet possibly revised by “wet” periglaciation at others. shape and scale broadly mirrors that of the CSPs de- 1) CSP formation on Earth is well constrained, de- scribed above. rived from extensive observations of thermokarst-lake Where the hydraulic potential of liquid water is drainage and alas formation [1]. thought to be the result of downslope and inter- The Moreaux-region mounds occur in topographic permafrost migration from elevated areas adjacent to lows. In principle, this facilitates the localized pooling the OSPs, the permafrost at the OSP locations is thin of near-surface meltwater and ice-core development by and discontinuous [e.g. 1] (Figs. 4a-c). This facilitates permafrost aggradation, heightened pore-water pres- the re-emergence of migrating water where the OSPs sure and freezing. Ice-core water origin could have form. Here the permafrost may or may not be been meteoric, albeit icy, and thaw-freeze cycling polygonised and need not be ice-rich, i.e. permafrost would have migrated the meltwater into the regolith. dominated by segregation ice [14]. A commonplace Widespread polygonisation and the ubiquitous incision observation: cracks form and radiate from the mound of the polygonised terrain by wavy depressions may be summit in response to tensile stress increases on the indicative of water having undergone freeze-thaw cy- sedimentary overburden; eventually the cracks may cling at the mound sites [9]. evolve into summit depressions [e.g. 14-15]. 2) With regard to terrestrial OSPs, ice-core origin has been hypothesised largely by geochemistry and the relative comparisons of meteoric, marine and/or juve- nile water [e.g. 14-16]. However, the plotting of actual hydro-geological pathways that induce the develop- ment of OSPs is absent from the literature. Assuming that the Moreux mounds are CSPs, the (c) origin of ice-core water could be: a) artesian and deep- ly seated; or b) meteoric and basal, sourced from prox- imal glacial-like landforms and delivered downslope to the mound locations by near-surface fractures. References: [1] Mackay, J.R. 1998. Géographie phy- sique et quaternaire 52, 3, 1-53. [2] Burr, D.M. et al. 2005. Fig. 4. a) Un-named open-system (valley) pingo on an outwash Icarus 178 (1), 56-73, doi.org/10.1016/2005.04.012. [3] plain, Axel Heiberg Island, Canada. Note, polygonised terrain in Soare, R.J. et al. 2005. Icarus 174, 373-382, doi.org/10.1016 the foreground. b) Pingo remnants on valley bottom (approx.. /j.icarus.2004.11.013. [4] Dundas, C.M. et al. (2008). Ge- 600 m wide), Central West-Greenland [16]. c) Plan view, Axel ophys. Res. Lett. 35, L04201, doi.org/10.1029/2007GL0317 Heiberg pingo (in oval) near Müller ice cap terminus (National 98. [5] Balme, M.R., Gallagher, C. 2009. EPSL 285 1-15, Air Photo Library, Ottawa, Canada). d) Terminus as seen from doi:10.1016/j.epsl.2009.05.031. [6] De Pablo, M.A., Komat- the pingo summit. Image credits for panels a and d: R. Soare. su, G. 2009. Icarus 199 (1), 49-74, doi.org/10.1016/j.icarus. 2008.09.007. [7] Soare, R.J. et al. 2013. Icarus 225 (2), 971- Alternatively, the hydraulic potential may be the 981. doi.org/10.1016/j.icarus.2012.08.041. [8] Soare, R.J. et work of sub-permafrost and deeply-seated (artesian) al. 2014. Icarus 225, 2, 971-981, doi.org/10.1016/j.icarus.01 groundwater. The groundwater upwells and migrates 2.08.041. [9] Soare et al. 2019. Icarus, in press. [10] Cos- through local geological faults and is sufficient in pres- tard, F., Kargel, J.S. 1995. Icarus 114, 93-112. [11] Mor- sure to uplift or break through continuous permafrost. genstern, A. et al. 2007. JGR 112, E06010, doi:10.1029/200 Here too, the surface permafrost may or may not be 6JE002869. [12] Séjourné, A. et al. 2011. PSS 59, 412-422, polygonised and need not be ice-rich [15-17]. doi:10.1016/j.pss.2011.01.007. [13] Mustard, J.F. et al. A third hypothesis suggests that near-surface faults 2001. Nature 412, 411-414, doi:10.1038/35 086515. [14] Müller, F. 1963. Technical Report 1073, NRC Canada. [15] deliver meltwater from the temperate basal-zones of Scholz, H., Baumann, M. 1977. Geol. of Greenland Survey glaciers through to the foregrounds or outwash plain Bull. 176, 104-108. [16] Christiansen, H.V. 1995. Danish J. sites where the OSPs form [17] (Fig. 4a,c). of Geog. 95, 42-47. [17] French, H.M. 2007. The periglacial environment. England: John Wiley & Sons. .
Details
-
File Typepdf
-
Upload Time-
-
Content LanguagesEnglish
-
Upload UserAnonymous/Not logged-in
-
File Pages2 Page
-
File Size-