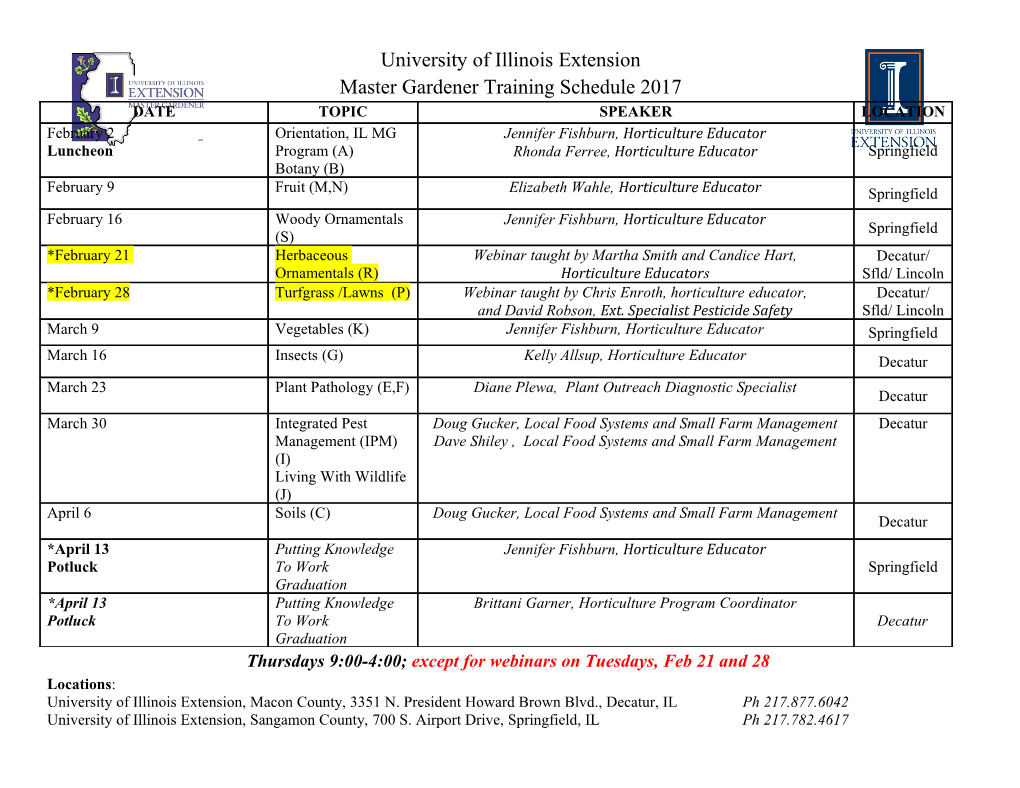
Ex vivo binding of the agonist PET radiotracer [11C]-(+)-PHNO to dopamine D2/D3 receptors in rat brain: Lack of correspondence to the D2 receptor two-affinity-state model by Patrick Neil McCormick A thesis submitted in conformity with the requirements for the degree of Doctor of Philosophy, Graduate department of Institute of Medical Science, in the University of Toronto © Copyright by Patrick Neil McCormick (2010) Ex vivo binding of the agonist PET radiotracer [11C]-(+)-PHNO to dopamine D2/D3 receptors in rat brain: Lack of correspondence to the D2 receptor two-affinity-state model Patrick Neil McCormick Doctor of Philosophy Institute of Medical Science University of Toronto 2010 Abstract The dopamine D2 receptor exists in vitro in two states of agonist affinity: a high-affinity state mediating dopamine’s physiological effects, and a physiologically-inert low-affinity state. Our primary goal was to determine the in vivo relevance of this two-affinity-state model for the agonist PET radiotracer [11C]-(+)-PHNO, developed for measurement of the D2 high-affinity state. Our second goal was to characterize the regional D2 versus D3 pharmacology of [3H]-(+)- PHNO binding and assess its utility for measuring drug occupancy at both receptor subtypes. Using ex vivo dual-radiotracer experiments in conscious rats, we showed that, contrary to the two-affinity-state model, the binding of [11C]-(+)-PHNO and the antagonist [3H]-raclopride were indistinguishably inhibited by D2 partial agonist (aripiprazole), indirect agonist (amphetamine) and full agonist ((-)-NPA) pretreatment. Furthermore, ex vivo [11C]-(+)-PHNO binding was unaffected by treatments that increase in vitro high-affinity state density (chronic amphetamine, ethanol-withdrawal), whereas unilateral 6-OHDA lesion, which increases total D2 receptor expression, similarly increased the ex vivo binding of [11C]-(+)-PHNO and [3H]- raclopride. These results do not support the in vivo validity of the two-affinity-state model, suggesting instead a single receptor state for [11C]-(+)-PHNO and [3H]-raclopride in conscious rat. Importantly, we also demonstrated that the increased amphetamine-sensitivity of the agonist ii radiotracers [11C]-(+)-PHNO and [11C]-(-)-NPA, commonly seen in isoflurane-anaesthetized animals and cited as evidence for the two-affinity-state model, is due to the confounding effects of anaesthesia. Using in vitro and ex vivo autoradiography in rat and the D3 receptor-selective drug SB277011, we found that [3H]-(+)-PHNO binding in striatum and cerebellum lobes 9 and 10 was due exclusively to D2 and D3 receptor binding, respectively, but in other extra-striatal regions to a mix of the two receptor subtypes. Surprisingly, the D3 contribution to [3H]-(+)- PHNO binding was greater ex vivo than in vitro. Also surprising, several antipsychotic drugs, at doses producing 80% D2 occupancy, produced insignificant (olanzapine, risperidone, haloperidol) or small (clozapine, ~35%) D3 occupancy, despite similarly occupying both receptor subtypes in vitro. These data reveal a significant discrepancy between in vitro and ex vivo measures of dopamine receptor binding and suggest that the D3 occupancy is not necessary for the therapeutic effect of antipsychotic drugs. iii Acknowledgments I would first like to thank Dr. Philip Seeman, who co-supervised my MSc degree and a portion of my Ph.D. project, for providing me with the opportunity to come to Toronto and begin my career in brain research. Nearing the end of my PhD, I am convinced that this is the correct path for me, and I owe this realization to the opportunity afforded me by Dr. Seeman. Had it not been for him, I might have ended up taking the offer to do a Ph.D. studying beetle pheromones, and not met any of my current colleagues, my wife or many of my dearest friends. I would also have learned nothing of the fascinating field of PET imaging (We’re pushing resolution limits with rodent, not to mention beetle scanning!). In terms of practical supervision and day-to-day guidance, my gratitude goes to Dr. Alan Wilson. Thanks, Alan, for providing me with an environment of rigourous, disciplined, logical investigation that has set a high standard in my mind for the way science should be conducted. Thank you also for your steadfast support throughout my studies, particularly when I found myself in the unenviable position of being part-way through a PhD without official supervision! On a personal note, your unflinching candour, which at first I found slightly intimidating, has become the characteristic I admire most in you. I doubt that the phrase “what you see is what you get” applies more aptly to any other sentient being in the universe. Thanks for your honesty, support and exceptional training. I would also like to thank many other people at the CAMH. To the director of the PET centre, Dr. Sylvain Houle, my sincere thanks for providing much-needed salary support when I found myself “out in the cold,” and for allowing me a comfortable space to work and learn. Thanks to the members of my PhD committee, Drs. Neil Vasdev, Jeff Meyer, Gary Remington, Shitij Kapur and Nathalie Ginovart, for helpful criticism, intellectual stimulation and continual encouragement. Thanks also, Neil, for involving me in various interesting projects, both at CAMH and elsewhere. I took it as a vote of confidence from an excellent scientist. Thanks to iv Armando Garcia, Winston Stableford, and Min Wong for the unfailing and punctual synthesis of [11C]-(+)-PHNO, without which this work would have been impossible, and to Doug Hussey, Jun Parkes, Alvina Ng, Greg Reckless, Zoe Rizos, Roger Raymond, Dr. José Nobrega and Mikael Palner for training me in the various techniques this work required and for their countless hours of help with experiments. Thanks to Lori Dixon for her strict yet flexible approach to animal facility matters, which tended to facilitate, rather than impede, the work in this thesis. Thanks to Isabelle Boileau for creating the parametric maps in the front of this thesis and for letting me participate in her [11C]-(+)-PHNO study (not to mention the decent monetary compensation). Thanks to the Canadian Institutes for Health Research for funding this work (grant numbers MOP-74702 and MOP-44051). I would also like to offer a line to the animals used in this work who, by virtue of fate, contributed far more to this project than anyone else. To my parents, thanks for your love and support, which I felt even during my (often spectacular) failures, for instilling me with a love of learning and nurturing my fascination with the world around me. To my brother, Andrew, and my sister, Jenn, thanks for sticking with me and wanting the best for me. Thanks to my late brother, Jeff, whose life was the single most powerful influence in mine. Thanks to Dr. Solomon Shapiro for his guidance and especially for his genuine love of Homo sapiens. Finally, special thanks to my wife, Conny, for continually shining a brilliant, clarifying light on the world, and for being an endless source of fun, humour and warmth I couldn’t have done without. v During my PhD, [11C]-(+)-PHNO often occupied my thoughts, but on a couple of occasions it even occupied my dopamine receptors! Shown above are parametric maps of [11C]-(+)-PHNO binding potential (BPND) in my brain at baseline (left) and after 35 mg oral amphetamine (right). In the top images, the greatest binding is seen in the striatum (here at a level including the caudate, putamen and globus pallidus) and in the bottom images within the substantia nigra. vi Table of contents 1. General introduction 1 2. Literature review 3 2.1. The dopaminergic system 4 2.1.1. Overview of the functional anatomy of the dopaminergic system 4 2.1.2. Intracellular effects of dopamine receptor activation 11 2.1.2.1. Intracellular effects of the D1-like receptor family 11 2.1.2.2. Intracellular effects of the D2-like receptor family 13 2.1.3. Brain distribution of dopamine receptors and the dopamine transporter 16 2.1.3.1. Distribution of the dopamine D1 receptor 16 2.1.3.2. Distribution of the dopamine D2 receptor 17 2.1.3.3. Distribution of the dopamine D3 receptor 19 2.1.3.4. Distribution of the dopamine D4 receptor 22 2.1.3.5. Distribution of the dopamine D5 receptor 23 2.1.3.6. Distribution of the dopamine transporter 24 2.1.4. Involvement of the dopaminergic system in substance abuse and schizophrenia 26 2.1.4.1. The dopaminergic system and substance abuse 26 2.1.4.2. The dopaminergic system and schizophrenia 29 2.2. Quantification of in vivo PET and SPECT radiotracer binding 34 2.2.1. Radiotracer binding under equilibrium conditions 34 2.2.2. Radiotracer binding under non-equilibrium conditions 42 2.2.2.1. Kinetic modeling of dynamic time-concentration data 43 2.2.2.2. Graphical analysis of dynamic time-concentration data 48 2.3. PET and SPECT radiotracers for dopaminergic imaging in human brain 53 2.3.1. Aromatic amino acid decarboxylase (AAAD), dopamine synthesis and storage 53 2.3.2. The dopamine transporter (DAT) 54 2.3.3. Vesicular monoamine transporter 2 (VMAT2) 58 2.3.4. Dopamine D1 receptors 58 2.3.5. Dopamine D2/D3 receptors 60 2.3.6. D2/D3 radiotracer-based imaging of endogenous dopamine 62 2.4. The high-affinity state of the dopamine D2 receptor and the development of agonist D2/D3 positron emission tomography radiotracers 66 2.4.1. [11C]-(-)-NPA 68 2.4.2. [11C]-(-)-MNPA 70 2.4.3. [11C]-(+)-PHNO 72 3. Brief introduction and rationale for thesis studies 79 4. Dopamine D2 receptor radiotracers [11C](+)-PHNO and [3H]raclopride are indistinguishably inhibited by D2 agonists and antagonists ex vivo 81 4.1.
Details
-
File Typepdf
-
Upload Time-
-
Content LanguagesEnglish
-
Upload UserAnonymous/Not logged-in
-
File Pages221 Page
-
File Size-