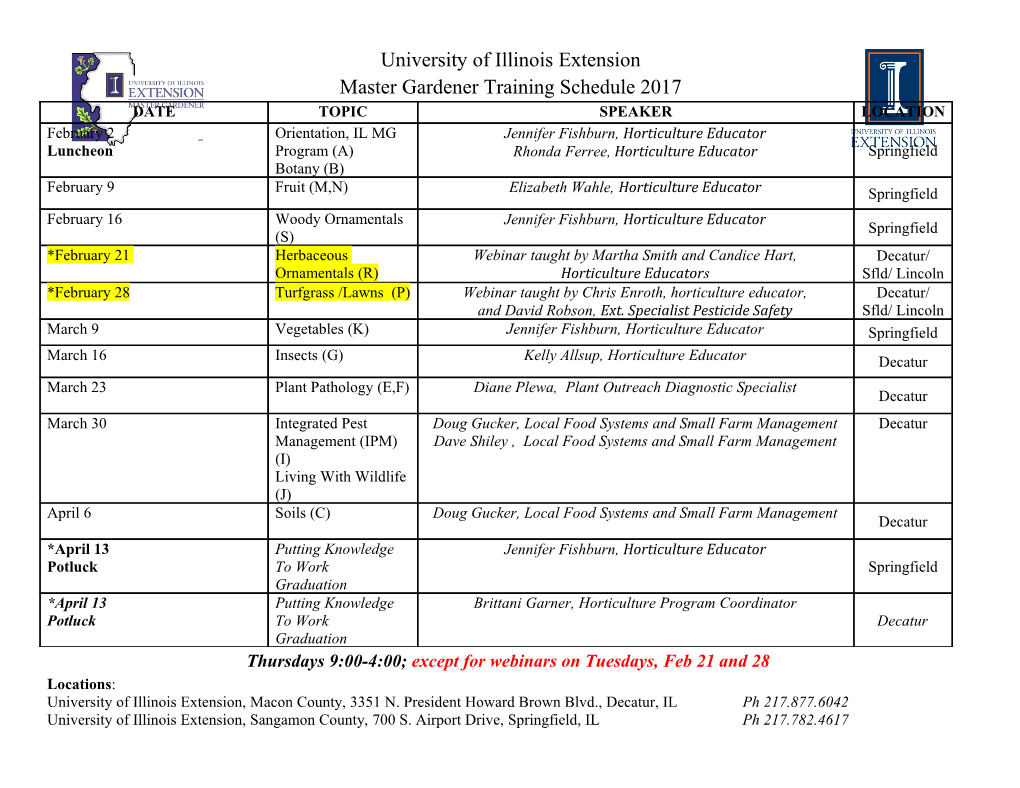
Atmospheric Ice Formation Processes 10 Bernd Ka¨rcher Abstract This contribution provides a basic introduction to the formation of ice in clouds. Various pathways to ice nucleation and factors controlling ice formation processes are addressed. Challenges in characterizing the atmospheric ice phase and novel approaches to better understanding the fundamental mechanisms involved in ice nucleation are outlined. 10.1 Introduction Water is the only material that occurs in all three phases in the Earth system. Water vapor is the most effective greenhouse gas and has a considerable effect on the radiation budget. A fundamental constraint on the abundance of water vapor is the Clausius-Clapeyron relationship (Becker 1978) determining the maximum amount of water vapor a given volume of air can hold in thermodynamic equilibrium. Saturation denotes the condition in which the vapor pressure of a substance in a condensed phase is equal to its equilibrium vapor pressure (see Fig. 10.1 for water). Water vapor in excess of the saturation values is termed supersaturated and the excess vapor turns into liquid water or ice. Liquid water is the main component in cloud droplets and most aerosol particles, allowing trace species to dissolve, forming aqueous solution droplets and initiating chemical reactions. Solid water in the form of ice crystals often initiates the formation of precipitation B. Kärcher (&) DLR, Institute of Atmospheric Physics (IPA), Münchner Straße 20, 82234 Oberpfaffenhofen, Germany e-mail: [email protected] U. Schumann (ed.), Atmospheric Physics, Research Topics in Aerospace, 151 DOI: 10.1007/978-3-642-30183-4_10, Ó Springer-Verlag Berlin Heidelberg 2012 152 B. Ka¨rcher 3 2 ratio 1 4 10 liquid water hexagonal ice 2 10 0 10 -2 10 mixed PMC PSC Ci phase -4 10 saturation vapor pressure/hPa -6 10 -150 -120 -90 -60 -30 0 o temperature/ C Fig. 10.1 Partial pressures of water molecules in thermodynamic equilibrium with liquid water and hexagonal ice, and their ratio (top panel), versus temperature (Murphy and Koop 2005). The spontaneous freezing temperature of supercooled pure water is indicated (square), along with the temperature ranges in which the various ice-containing clouds typically form. The water-to-ice pressure ratio, R, is indicated by a solid curve at temperatures where pure liquid water exists. Ice supersaturation (R–1), in mixed-phase clouds reaches a peak value of *0.47 at –40 °C. The thick solid curve marks the ice supersaturation where supercooled aqueous aerosol droplets freeze homogeneously in cirrus and PSCs (Koop 2004) in tropospheric clouds and is involved in cloud electrification. High altitude clouds composed entirely of ice crystals warm the climate by absorbing Earth’s infrared radiation (cirrus and contrail cirrus clouds, altitudes *10 km), are involved in polar ozone depletion (polar stratospheric clouds, PSCs, *20 km), and act as sensitive indicators of atmospheric changes (polar mesospheric clouds, PMCs, *85 km). Ice is the thermodynamically most stable water phase at sub- freezing temperatures. Ice nucleation plays an important role in diverse areas such as cryobiology and atmospheric cloud formation. In general, nucleation summarizes the processes by which material is transferred from one phase to another. The formation of a new phase, initiated by an external forcing, is driven by a supersaturation of the parent phase (vapor or liquid)—making it more abundant than dictated by thermody- namic equilibrium (i.e., in saturated conditions). The forcing, usually a cooling, is necessary to overcome the energy barrier to nucleation. The parent phase is unstable, exhibiting a tendency to establish saturated (equilibrium) conditions. The new phase has lower bulk energy and is therefore more stable. Once molecular- sized, stable patches of the new phase (‘critical clusters’) have been created due to 10 Atmospheric Ice Formation Processes 153 the energy supplied by thermal molecular motion, they grow further, depleting the parent supersaturation. In the case of liquid water/ice particles, this latter growth process is termed condensation/deposition; the reverse processes are evaporation/ sublimation. A water phase is ice-supersaturated at a certain temperature if the concentration of water molecules exceeds the ice-saturated value. In Earth’s atmosphere, ice forms in ice-supersaturated conditions mainly within liquid water or aqueous aerosol droplets, or on the solid surfaces of a subset of aerosol particles known as heterogeneous ice nuclei (IN). Ice crystals formed this way acquire sizes ranging between a millionth of a centimeter (0.01 lm) and several millimeters (1 000 lm) and can develop into precipitation particles (snow, graupel, hail) in low-altitude clouds. It has long been known that the geometrical forms of snowflakes bear evidence of the hexagonal crystal lattice of water molecules that are tied together by a hydrogen-bonding network.1 Three geometrical arrangements of ice exist in the atmosphere: hexagonal, cubic, and amorphous (noncrystalline) (Hobbs 1974). The two latter form at low temperatures and transform into the more stable, hexagonal, arrangement upon warming. Water ice has also been discovered on other planets in our solar system, on some of their moons, in the rings of Saturn, and on asteroidal surfaces. Hexagonal or cubic ice does not nucleate from the vapor phase alone in the atmosphere. Therefore, aerosol particles must serve as the precursors of ice crystals. This chapter provides a brief overview of aerosol-mediated pathways to ice formation in clouds (nucleation modes) and addresses dynamical controls of cloud ice formation with special emphasis on cirrus. 10.2 Aerosol-Mediated Modes of Ice Nucleation Cloud particles form by nucleation (i.e., nonequilibrium) processes (Houze Jr. 1993). At temperatures below the equilibrium melting point (0 °C or 273.15 K) cloud particles may be composed of either supercooled water or ice. Ice crystals may form either homogeneously in cloud water droplets, in water condensed on aerosol particles, or heterogeneously involving water with solid impurities. The exact temperature at which ice forms depends on the nucleation process. The presence of IN in supercooled liquid water droplets has been shown to be necessary for the formation of ice-containing clouds at temperatures above about –30 °C. Incomplete understanding of ice initiation—the first appearance of the ice phase—is a large obstacle for the detailed numerical modeling of ice processes in clouds. 1 Water is a highly ordered liquid with water molecules conjoined by hydrogen atoms. The exact number of hydrogen bonds formed by a molecule of liquid water (up to four because the oxygen atom of one water molecule has two lone pairs of electrons) depends on the temperature. In crystalline ice, hydrogen bonding creates a lattice structure exhibiting long range order. Amor- phous water lacks this long range order yet it is still more ‘solid-like’ than liquid water. 154 B. Ka¨rcher 10.2.1 Homogeneous Ice Nucleation Small samples of liquid water can be supercooled to a temperature of about – 40 °C(&235 K), the spontaneous (or homogeneous) freezing temperature. Homogeneous freezing occurs at a range of temperatures depending on the sample volume (droplet size), the rate of cooling, the degree of purity of the water substance, and on other factors (Pruppacher and Klett 1978). The tem- perature regime in which supercooled liquid water droplets and ice crystals can coexist in the atmosphere defines the existence region of mixed-phase clouds (Fig. 10.1). Below the spontaneous freezing temperature, ice nucleates in aqueous droplets in a very short time (relative to meteorological time scales), quickly solidifying the entire droplet volume. In such cold conditions, water droplets can no longer exist. Cirrus and contrail cirrus are pure ice clouds occurring across a wide range of temperatures. Aqueous aerosol particles containing soluble matter (solutes) at high humidity are sometimes called ‘haze’ droplets. A common solute in atmospheric (particu- larly stratospheric) aerosol particles is sulfuric acid, but organic species are taken up by them as well. Hygroscopic material dissolved in aerosol particles attracts water molecules and thereby decreases the freezing temperature of pure water. In this way, ice nucleation can be suppressed to very low temperatures. Enhanced uptake of nitric acid in aqueous sulfuric acid particles causes the formation of liquid PSC particles in the winter polar lower stratosphere. These particles freeze homogeneously around –90 °C(&185 K), forming ice-phase clouds.2 PSCs are largely responsible for the formation of the ‘ozone hole’ (Solomon 1999). Solutes may precipitate in aqueous solution particles when the solute con- centration becomes too high, forming crystalline inclusions (e.g., in sea salt particles containing sodium chloride as solute). With increasing humidity hygroscopic solution particles absorb more water molecules and those precipi- tates can melt away. This solid-to-liquid phase transition is termed deliques- cence. Often deliquescence occurs before freezing. Homogeneous freezing temperatures of fully liquid, aqueous solution droplets have been measured below -40 °C for a wide array of solutes and solute concentrations (Koop 2004). It has been found that homogeneous ice nucleation in aerosols com- mences very suddenly within a narrow range of high ice supersaturations and is determined by the temperature and water activity—a thermodynamic property of the solution
Details
-
File Typepdf
-
Upload Time-
-
Content LanguagesEnglish
-
Upload UserAnonymous/Not logged-in
-
File Pages17 Page
-
File Size-