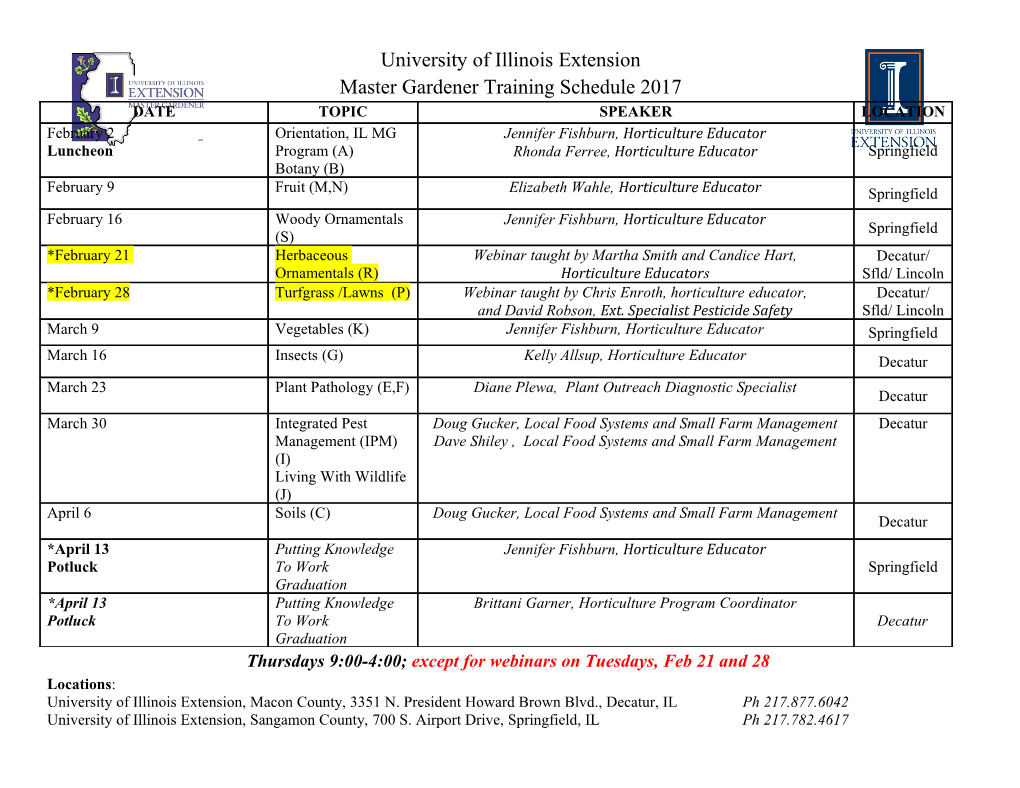
bioRxiv preprint doi: https://doi.org/10.1101/695544; this version posted July 8, 2019. The copyright holder for this preprint (which was not certified by peer review) is the author/funder, who has granted bioRxiv a license to display the preprint in perpetuity. It is made available under aCC-BY-ND 4.0 International license. 1 Visual pigment evolution in Characiformes: the dynamic interplay of teleost 2 whole-genome duplication, surviving opsins and spectral tuning. 3 4 Daniel Escobar-Camacho1, Karen L. Carleton1, Devika W. Narain2, Michele E.R. Pierotti3 5 6 1Department of Biology, University of Maryland, College Park, MD 20742, USA. 7 2Environmental Sciences, Anton de Kom University of Suriname, Paramaribo, Suriname. 8 3Naos Marine Laboratories, Smithsonian Tropical Research Institute, Panama, Republic 9 of Panama. 10 11 12 Corresponding author 13 Daniel Escobar-Camacho 14 [email protected] 15 16 17 18 19 20 21 22 23 24 25 26 27 28 29 30 31 32 1 bioRxiv preprint doi: https://doi.org/10.1101/695544; this version posted July 8, 2019. The copyright holder for this preprint (which was not certified by peer review) is the author/funder, who has granted bioRxiv a license to display the preprint in perpetuity. It is made available under aCC-BY-ND 4.0 International license. 33 Abstract 34 Vision represents an excellent model for studying adaptation, given the genotype-to- 35 phenotype-map that has been characterized in a number of taxa. Fish possess a diverse 36 range of visual sensitivities and adaptations to underwater light making them an 37 excellent group to study visual system evolution. In particular, some speciose but 38 understudied lineages can provide a unique opportunity to better understand aspects of 39 visual system evolution such as opsin gene duplication and neofunctionalization. In this 40 study, we characterized the visual system of Neotropical Characiformes, which is the 41 result of several spectral tuning mechanisms acting in concert including gene 42 duplications and losses, gene conversion, opsin amino acid sequence and expression 43 variation, and A1/A2-chromophore shifts. The Characiforms we studied utilize three cone 44 opsin classes (SWS2, RH2, LWS) and a rod opsin (RH1). However, the characiform’s 45 entire opsin gene repertoire is a product of dynamic evolution by opsin gene loss 46 (SWS1, RH2) and duplication (LWS, RH1). The LWS- and RH1-duplicates originated 47 from a teleost specific whole-genome duplication as well as characiform-specific 48 duplication events. Both LWS-opsins exhibit gene conversion and, through substitutions 49 in key tuning sites, one of the LWS-paralogs has acquired spectral sensitivity to green 50 light. These sequence changes suggest reversion and parallel evolution of key tuning 51 sites. In addition, characiforms exhibited species-specific differences in opsin 52 expression. Finally, we found interspecific and intraspecific variation in the use of A1/A2- 53 chromophores correlating with the light environment. These multiple mechanisms may 54 be a result of the highly diverse visual environments where Characiformes have evolved. 55 56 Introduction 57 To fully understand the evolutionary history of genes and their relevance for adaptation 58 and speciation, it is important to explore the genotype to phenotype map and how this 59 relates to the environment. Evolutionary studies of genes such as the ones involved in 60 the first steps of vision can provide valuable insights in the acquisition of new functions 61 and their adaptive significance. In vertebrates, vision starts when light reaches the retina 62 and is detected by rod (night vision) or cone (diurnal vision) photoreceptors. 63 Photoreceptors are packed with visual pigments that are composed of two components: 64 an opsin protein with seven α-helices enclosing a ligand-binding pocket, and a light- 65 sensitive chromophore, 11-cis retinal (Bowmaker 2008; Yokoyama 2008). There can be 2 bioRxiv preprint doi: https://doi.org/10.1101/695544; this version posted July 8, 2019. The copyright holder for this preprint (which was not certified by peer review) is the author/funder, who has granted bioRxiv a license to display the preprint in perpetuity. It is made available under aCC-BY-ND 4.0 International license. 66 multiple cone types containing different visual pigments that absorb light maximally in 67 different parts of the wavelength spectrum. 68 69 There are four classes of cone pigments encoded by opsin genes among vertebrates: a 70 short-wave class (SWS1) sensitive to ultraviolet-violet light (350-400 nm), a second 71 short-wave class (SWS2) sensitive to violet-blue (410-490 nm), a middle-wave class 72 (RH2) sensitive to green (480-535 nm), and a middle- to long-wave class (LWS) 73 sensitive to the green and red spectral region (490-570 nm) (Bowmaker and Hunt 2006; 74 Bowmaker 2008). All four cone classes are the product of a series of gene duplications 75 from an ancestral single opsin gene that appeared early in vertebrate evolution (450 76 MYA) (Bowmaker 1998; Bowmaker and Hunt 2006; Bowmaker 2008). This results in a 77 spectral tuning mechanism that is based on nucleotide variation: if a nucleotide 78 substitution leads to the replacement of an amino acid that alters the interaction of the 79 chromophore and the opsin, this will lead to a spectral shift in the maximal absorbance 80 (λmax) of the visual pigment. Consequently, variation in λmax between visual pigments is 81 the product of the interaction of different opsin classes and the identical 11-cis retinal. 82 The shift in λmax caused by a single amino acid substitution depends on the amino acid 83 identity and site, with most causing smaller shifts (2-10 nm) and a few sites causing very 84 large shifts (e.g. 75 nm) (Yokoyama 2008). 85 86 Among vertebrates, fish are ideal for the study of visual pigment evolution. First, 87 because of the physico-chemical properties of water, this medium has a profound effect 88 on light transmission. Water absorbs and scatters much of the incoming light, and this 89 inevitably causes great variation across aquatic habitats that differ in concentrations of 90 particulates and dissolved compounds (Loew and McFarland 1990; Warrant and 91 Johnsen 2013). This results in several adaptations in fish visual systems. Second, due to 92 their phylogenetic history, species richness, diverse ecologies, and diverse spectral 93 sensitivities, teleosts offer an excellent system for studying the evolution of visual 94 pigments. Spectral sensitivities have been documented for quite a few fish species 95 (Schwanzara 1967; Muntz 1973; Levine and MacNichol 1979; Bowmaker et al. 1994; 96 Lythgoe et al. 1994; Carleton 2009) and the dynamic evolution of the different opsin 97 classes has been actively studied (Bowmaker 2008; Yokoyama 2008; Hofmann and 98 Carleton 2009; Davies et al. 2012; Rennison et al. 2012; Cortesi et al. 2015; Lin et al. 99 2017; Musilova et al. 2019). 3 bioRxiv preprint doi: https://doi.org/10.1101/695544; this version posted July 8, 2019. The copyright holder for this preprint (which was not certified by peer review) is the author/funder, who has granted bioRxiv a license to display the preprint in perpetuity. It is made available under aCC-BY-ND 4.0 International license. 100 101 Characiformes, with more than 2000 described species, is an extremely diverse group of 102 freshwater fishes inhabiting a wide range of ecosystems. This order includes at least 23 103 families with dozens of species being described each year (Oliveira et al. 2011; Arcila et 104 al. 2018; Froese and Pauly 2019). Their Gondwanan origin, wide distribution, species 105 richness and colorful patterns, make them an ideal group for studying the evolution of 106 their visual system and its adaptation to the light environment. Data on the opsin 107 repertoire of Characiformes has only been reported for the tetra Astyanax fasciatus 108 (Yokoyama and Yokoyama 1990a; Yokoyama and Yokoyama 1993; Register et al. 109 1994; Yokoyama et al. 1995; Yokoyama et al. 2008). These studies characterized its 110 visual pigments and showed how this species has a duplication in the LWS opsin in 111 which one copy became sensitive to green light through amino acid substitutions; a 112 remarkable example of convergent evolution with green sensitivity in humans 113 (Yokoyama and Yokoyama 1990a). Recent studies have analyzed the origins of 114 Astyanax opsin genes more in depth and concluded that these duplicates are surviving 115 opsins from the teleost-specific genome duplication (TGD) (300-450 MYA: Taylor et al. 116 2001; Meyer and Peer 2005; Liu et al. 2018). 117 118 In this study, we expand the molecular characterization of the visual system in 119 Characiformes. We showcase the complex evolutionary dynamics of their opsin gene 120 repertoire and we examine the diverse set of spectral tuning mechanisms present in this 121 group. 122 123 Results 124 Opsin gene sequences 125 Opsin complements 126 Through phylogenetic analyses of 15 characiform species, we identified fully functional 127 sequences belonging to three cone opsin classes (SWS2, RH2, LWS) as well as the rod 128 opsin (RH1) (Fig. 1-2, Fig S1-3). The opsin-gene set within Characiformes seems highly 129 variable because we found variation in the presence/absence of some opsins as well as 130 several duplications (Fig. 3). We did not find sequences belonging to the UV-light 131 sensitive opsin (SWS1), either in the transcriptomes or genomes (Fig. S1). We also did 132 not detect the RH2 opsin in the transcriptomes of C. spilurus, H. microlepis, S. 4 bioRxiv preprint doi: https://doi.org/10.1101/695544; this version posted July 8, 2019. The copyright holder for this preprint (which was not certified by peer review) is the author/funder, who has granted bioRxiv a license to display the preprint in perpetuity.
Details
-
File Typepdf
-
Upload Time-
-
Content LanguagesEnglish
-
Upload UserAnonymous/Not logged-in
-
File Pages37 Page
-
File Size-