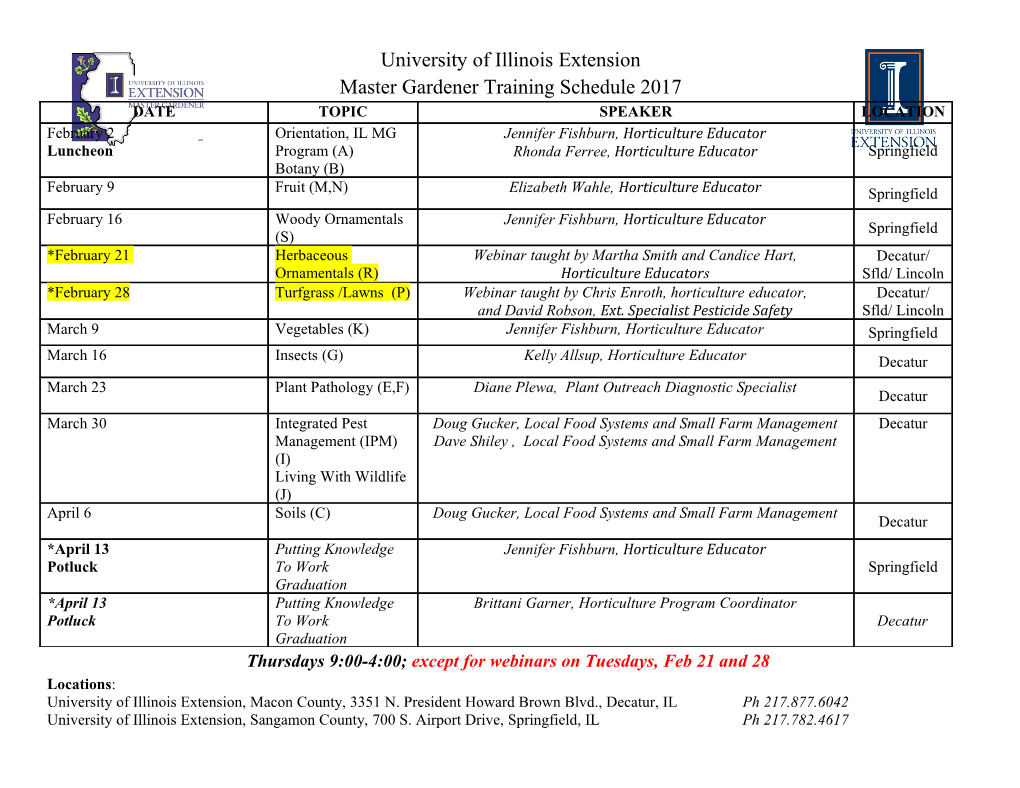
Vol 1, No 2, 2020, 223-234 DOI: 10.22044/rera.2020.9521.1028 Turbocharged Diesel Engine Power Production Enhancement: Proposing a Novel Thermal-Driven Supercharging System based on Kalina Cycle 1 1 1 F. Salek , A. Eskandary Nasrabad and M.M. Naserian * 1- Department of Mechanical Engineering, Faculty of Montazeri, Khorasan Razavi Branch, Technical and Vocational University (TVU), Mashhad, Iran. Receive Date 3 April 2020; Revised 26 April 2020; Accepted Date 25 May 2020 *Corresponding authors: [email protected] (M. M. Naserian) Abstract In this paper, a novel thermal-driven supercharging system is proposed for downsizing of a turbocharged diesel engine. Furthermore, the Kalina cycle is used as a waste heat recovery system to run the mounted supercharging system. The waste heat of air in engine exhaust and intake pipes is converted to the cooling and mechanical power by the Kalina cycle. The mechanical power produced by the Kalina cycle is transferred to an air compressor to charge extra air to the engine in order to generate more power. This feature can be used for downsizing the turbo-charged heavy duty diesel engine. In addition, the heat rejected from the engine intercooler is transferred to the Kalina cycle vapor generator component, and part of the engine exhaust waste heat is also used for superheating the Kalina working fluid before entering the engine. Then the first and second law analyses are performed to assess the operation of the engine in different conditions. Moreover, an economic model is provided for the Kalina cycle, which is added to the engine as a supplementary component. Finally, the simple payback and net present value methods are used for the economic evaluation of the added supplementary system. According to the results obtained, mounting the novel waste heat-driven air charging system results in the incrementation of air mass flow rate, which leads to an extra power generation (between 9 kW and 25 kW). The payback period and the profitability index of the project are approximately 3.81 years and 1.26, respectively. Keywords: Waste heat recovery, Diesel engine, Kalina cycle, Power production, Cooling power. 1. Introduction Nowadays, the researchers are looking for ways to used to increase the vehicle driving force. decrease fuel consumption in the internal Recently, some other researchers have focused on combustion engines. One of the most efficient using the recovered energy to run other auxiliary ways to achieve this purpose is to use the waste equipment such as air charging systems [11, 12], heat recovery (WHR) systems. The which result in increasing the engine thermal thermodynamic cycles such as the Rankine cycles efficiency and the output power. [1-3], Brayton cycle [4, 5], and refrigeration cycles There are some researchers who have studied [6, 7] are used to recover the engine waste heat to engine waste heat recovery by employing the other usable types of energy such as mechanical, Rankine cycle and transfer generated power to the electrical, and cooling energy. In some research engine supercharging system [15, 16]. Alessandro works, the thermo-economic analysis of different Romagnoli et al. [15] have investigated the benefits types of multi-generation systems that contain of coupling an organic Rankine cycle, which various waste heat recovery systems has been absorbs heat from engine exhaust to engine performed for evaluation of their performance [8- supercharger. During their research work, it was 10]. found that the implement of this technology on The recovered power is utilized in many ways. For vehicles would decrease the engine BSFC by instance, in the turbo-compounding systems [11- approximately 5%. However, other 14], the power generated by the WHR systems is thermodynamic cycles such as the Brayton and transferred to the engine driveline. Therefore, it is Kalina cycles, which are widely used as WHR F. Salek et al./ Renewable Energy Research and Application, Vol 1, No 2, 2020, 223-234 systems [17], have not been examined yet as a the Kalina cycle working fluid. The output power source of power for the mentioned air of Turb2 is transferred mechanically to Comp1, supercharging system. which acts as a supercharger in the air charging In this work, a novel waste heat driven system. It leads to more power and less soot supercharging system was analyzed using the productions [18, 19]. thermo-economic and exergy analysis method. The It can be figured out from figure 2 that two heat Kalina cycle was employed to generate power from exchangers (HEX2 and HEX3) are mounted in the engine waste heat to energize the engine air engine exhaust system. HEX2 is used to produce charging system. The waste heat of air going hot water for domestic or other activities. However, through the engine intercooler and exhaust is it does not absorb all the available heat in flue transferred to the Kalina cycle and is converted to gases, so HEX3 is employed to transfer the the cooling and mechanical power. The mechanical available heat to the Kalina cycle for superheating power is used to run a supercharger, which is ammonia. mounted before air compressor in engine intake As mentioned earlier, the Kalina cycle absorbs heat line. Thus the Kalina cycle working fluid absorbs from two sources: engine air charging system and air heat at engine intercooler, which results in exhaust system. Then the waste heat is converted reducing the air temperature. Therefore, extra air to the cooling and mechanical power, which is used would be inhaled by the engine. The produced to drive a supercharger. The cooling power can be cooling power can be used for vehicle air used to produce cold water for domestic use or for conditioning system or produce cold water. air conditioning systems. The technical specifics of the mentioned turbo- 2. System description charged diesel engine are provided in table 1. The provided system consists of three components: air charging system, engine, and exhaust system and Kalina cycle. The air charging system contains Table 1. Specifications of the proposed turbocharged two air compressors (Comp1 and Comp2) and a diesel engine [20]. Parameter Unit Value heat exchanger (HEX1) that decreases the Manufacturer - John Deere temperature of air. In fact, some of the engine inlet Engine model - 6068TF250 air heat is absorbed by HEX1 and transferred to the Engine type - 4Cycle,Turbocharged Cylinder arrangement - 6 Inline Kalina cycle. Therefore, by employment of HEX1, Displacement Volume L 6.79 two achievements can be made: cooling down air Bore mm 106 Stroke mm 127 temperature leading to inhaling extra air by engine, Compression ratio 17 and transferring air heat to the WHR system. Rated RPM rpm 1800 Maximum power at Comp2 and Turb1 are coupled to each other as the kW 142 main components of the engine turbocharging rated RPM system. Turb2 is a steam turbine that is rotated by Figure 1. Energy flow diagram of system. 224 F. Salek et al./ Renewable Energy Research and Application, Vol 1, No 2, 2020, 223-234 Figure 2. A block diagram of the whole system 3. Mathematical modeling 3.1.1. Diesel engine A turbocharged diesel engine includes two external 3.1. Energy modeling and four internal thermodynamic processes. Due to providing the first law analysis system, Applying the first law of thermodynamic for each mass conservation, concentration, and energy process of engine [24-26]: balance equations are applied to each component of the system, which are considered as control volume 3.1.1.1. Air charging system [21-23]. Air compressors in the air-charging system: - mass conservation: 푊̇푐표푚푝 = 푚̇ 푎(ℎ푒 − ℎ) (4) ∑ 푚̇ = ∑ 푚̇ 푒 (1) ℎ푖푠푒.푒−ℎ푖 - energy equation: 휂푠푒.푐표푚푝 = (5) ℎ푒−ℎ푖 ∑ 푄̇ + ∑ 푊̇ = ∑ 푚̇ 푒ℎ푒 − ∑ 푚̇ ℎ (2) Intercooler in the air-charging system: - concentration equation: 푄̇퐻퐸푋1 = 푚̇ 푎(ℎ − ℎ푒) (6) ∑ 푚̇ 푋 = ∑ 푚̇ 푒푋푒 (3) 3.1.1.2. Engine internal processes Some assumptions are considered to simplify the equations: - Each component is considered as a control volume that can exchange work and heat with ambient - Thermodynamic equilibrium is applied to the whole system components - Steady conditions are assumed in all points of system - The pressure drop due to friction caused by the Figure 3. The T-S and P-V diagrams of the diesel cycle. fluid flow inside the components is denied 225 F. Salek et al./ Renewable Energy Research and Application, Vol 1, No 2, 2020, 223-234 Engine intake process: 푊̇ 표푢푡푝푢푡 푚 휂푡.푑푒푠푒푙 = (25) 퐴퐹 = 푎 (7) 푚̇ 푓퐿퐻푉 푚푓 푊 = 푊 + 푊 − 푊 − 푉 (푃 − 푃 ) (26) 푚×푁×푛 푛푒푡 푒푥푝1 푒푥푝2 푐푝 푑 푒1 푎4 푚̇ = 푐 (8) 2×60 푊 ×푁×푛 ×휂 ×휂 푊̇ = 푛푒푡 푐 푏 푒 (27) 푇1 = 푇푎4 (9) 표푢푡푝푢푡 2×60 푃 = 푃 (10) 푚̇ 푓 1 푎4 퐵푆퐹퐶 = (28) 푊̇ 표푢푡푝푢푡 푚푡표푡푎푙 = 푚푎 + 푚푓 (11) 3.1.1.3. Exhaust system 푉퐵퐷퐶 Heat exchangers: 푚푡표푡푎푙.푚푎푥 = (12) 푣1 푚̇ 푐(ℎ푒−ℎ푖) 휂퐻퐸푋 = (29) 푚푡표푡푎푙 ≤ 0.9푚푡표푡푎푙.푚푎푥 (13) 푚̇ 푒(ℎ푖−ℎ푒) Engine compression process: Turbine 1: ̇ 푊푐푝 = 푚푡표푡푎푙(푢2 − 푢1) (14) 푊푇푢푟푏1 = 푚̇ 푒(ℎ푒1 − ℎ푒2) (30) 푢푖푠푒.2−푢1 ℎ푖−ℎ푒 휂푠푒.푐푝 = (15) 휂푠푒.푡푢푟푏 = (31) 푢2−푢1 ℎ푖−ℎ푖푠푒.푒 푘 푃2 푊̇ × 휂 = 푊̇ (32) 푟푐 = (16) 푇푢푟푏1 푚 푐표푚푝2 푃1 3.1.2. Kalina Cycle Engine combustion process: In the Kalina cycle, NH3-H2O is employed as the Absorbing heat in a constant pressure process: working fluid. Applying the first law of thermodynamic, continuum, and concentration 푄푐표푚푏 = 푚푡표푡푎푙(ℎ3 − ℎ2) (17) equations for each stream of cycle [27-29]: Generator: Expansion in a constant pressure process: 푄̇퐻퐸푋1 = 푚̇ 푘3ℎ푘3 + 푚̇ 푘12ℎ푘12 − 푚̇ 푘7ℎ푘7 − 푄푐표푚푏 − 푊푒푥푝1 = 푚푡표푡푎푙(푢3 − 푢2) (18) 푚̇ 푘4ℎ푘4 (33) 푄푐표푚푏 = 푚푡표푡푎푙 × 퐿퐻푉 × 휂푐표푚푏 (19) Separator: Expansion process: 푄̇ = 푚̇ ℎ − 푚̇ ℎ − 푚̇ ℎ (34) 푆푒푝 푘7 푘7 푘12 푘12 푘8 푘8 푊 = 푚 (푢 − 푢 ) (20) 푒푥푝2 푡표푡푎푙 3 4 Heat exchanger 3: 푄푐표푚푏 = 푚푡표푡푎푙 × 퐿퐻푉 × 휂푐표푚푏 (21) 푄̇퐻퐸푋3 = 푚̇ 푘9ℎ푘9 − 푚̇ 푘8ℎ푘8 (35) 푢3−푢4 휂푠푒.푒푝 = (22) 푢3−푢푖푠푒.4 Turbine 2: 푃4 푣3 ̇ = [ ]푘 (23) 푊푇푢푟푏2 = 푚̇ 푘9ℎ푘9 − 푚̇ 푘10ℎ푘10 (36) 푃3 푣4 푊̇ × 휂 = 푊̇ (37) Exhaling process: 푇푢푟푏2 푚 푐표푚푝1 Evaporator: 푄 = 푚 (푢 − 푢 ) (24) 푒푥 푡표푡푎푙 4 5 ̇ Engine thermal efficiency and total power 푄퐸푣푎푝 = 푚̇ 푘10ℎ푘10 − 푚̇ 푘11ℎ푘11 (38) generation: 226 F.
Details
-
File Typepdf
-
Upload Time-
-
Content LanguagesEnglish
-
Upload UserAnonymous/Not logged-in
-
File Pages12 Page
-
File Size-