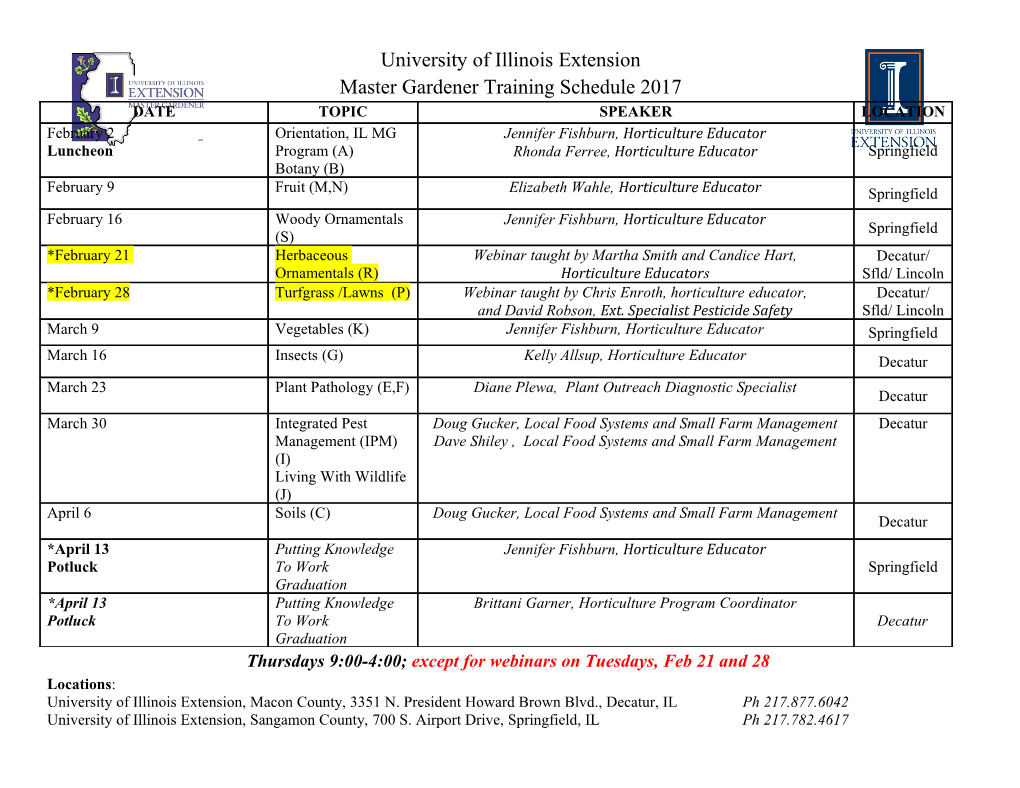
12 Antisense RNAs in Bacteria and Their Genetic Elements ∗ E. Gerhart H. Wagner Institute of Cell and Molecular Biology Biomedical Center Uppsala University 751 24 Uppsala, Sweden Shoshy Altuvia Department of Molecular Genetics and Biotechnology The Hebrew University—Hadassah Medical School 91120 Jerusalem, Israel Pascale Romby UPR 9002, CNRS-IBMC Institut de Biologie Moleculaire´ et Cellulaire 67084 Strasbourg Cedex, France I. Introduction—Antisense Principle and Gene Regulation II. Naturally Occurring Antisense RNA Control Systems III. Antisense RNA Mechanisms and Biological Context A. Plasmid Systems B. Transposons C. Bacteriophages D. Chromosomally Encoded Antisense Systems IV. Antisense Structure and Kinetics of Binding A. Antisense and Target RNA Structure B. Mutations Affect Binding Rate and Specificity C. RNA–RNA Binding Kinetics D. Binding Pathways and Topology Problems *To whom correspondence should be addressed: E-mail: [email protected]; Telephone: +46 18 4714866; Fax: +46 18 530396. Advances in Genetics, Vol. 46 361 Copyright 2002, Elsevier Science (USA). All rights reserved. 0065-2660/02 $35.00 362 Wagner et al. V. Matching Antisense RNA Properties with Biological Roles A. The Role of Structure B. Short or Long Half-Life C. Single or Multiple Targets D. Protein Involvement E. Beyond intuition and experiments VI. Concluding Remarks References ABSTRACT Antisense RNA-mediated regulation is widespread in bacteria. Most antisense RNA control systems have been found in plasmids, phages, and transposons. Fewer examples were identified in bacterial chromosomes. This chapter summa- rizes our current knowledge about antisense RNAs with respect to their occur- rence, their biological roles, and their diverse mechanisms of action. Examples of cis- or trans-encoded antisense RNAs are discussed, and their properties com- pared. Most antisense RNAs are posttranscriptionally acting inhibitors of target genes, but a few examples of activator antisense RNAs are known. The implica- tions of RNA structure on topologically and kinetically favored binding pathways are addressed, and solutions that have evolved to permit productive interactions between intricately folded RNAs are discussed. Finally, we describe how particu- lar properties of individual antisense/target RNA systems match their respective biological roles. C 2002, Elsevier Science (USA). I. INTRODUCTION—ANTISENSE PRINCIPLE AND GENE REGULATION Homology effects—in a broad sense—underlie a variety of effects that alter gene expression in all organisms. The common denominator in all these diverse biologi- cal phenomena is some form of interaction between nucleic acids, based either on homology/sequence similarity or on complementarity. Antisense RNAs are a class of regulators of gene expression that act on target RNAs via sequence comple- mentarity. In the vast majority of the known cases this entails inhibition of target RNA function. In a few cases, antisense RNAs can activate gene expression. In other cases, in particular in eukaryotes, a variety of processes make use of RNA sequence complementarity to mediate or facilitate biological processes (RNA edit- ing, splicing, etc.). However, in the context of this article, a bona-fide antisense RNA is an independent regulator of one or more target genes, whose effect is 12. Antisense RNA in Bacteria 363 exerted posttranscriptionally. Furthermore, we will be concerned only with sys- tems in which antisense RNAs play biological roles in nature, and disregard all cases in which antisense RNAs have artificially been introduced to interfere with gene expression. Following this definition, very few cases in eukaryotes (Hildebrandt and Nellen, 1992; Reinhart et al., 2000; Wightman et al., 1993) and archea (Stolt and Zillig, 1993a) qualify, while the overwhelming majority of natural antisense RNA-regulated systems are found in bacteria, in particular in their accessory ge- netic elements—plasmids, phages, and transposons (Wagner and Simons, 1994). From the examples of bacterial antisense RNAs known, it can be concluded that they are generally small (most often 55–150 nt long), diffusible, and untranslated. They are in addition characterized by distinct secondary and tertiary structures, and particular motifs appear to correlate with regulatory performance (Figure 12.1, see color insert). Contrary to earlier concepts, our current understanding is that the high specificity and efficiency displayed by antisense RNA control systems cannot be explained by simple sequence complementarity-dependent hybridiza- tion, but is rather a consequence of interactions between intricately designed three-dimensional structures of the interactant RNAs. This review will focus on the biology of a number of well-characterized bacterial systems, give an overview of the mechanisms of action used by anti- sense RNAs, discuss the particular properties of antisense RNAs with respect to structure, binding kinetics, and topology, and set some characteristic features in context with their biological functions. Reviews on some aspects of bacterial an- tisense RNA have been published previously (Delihas, 1995; Eguchi et al., 1991; Nordstrom¨ and Wagner, 1994; Wagner and Brantl, 1998; Wagner and Simons, 1994; Zeiler and Simons, 1998). II. NATURALLY OCCURRING ANTISENSE RNA CONTROL SYSTEMS The first natural antisense RNAs were discovered in 1981. Tomizawa and co-workers showed that a small, plasmid-encoded regulator controlled the copy number of a plasmid, ColE1 (Tomizawa and Itoh, 1981; Tomizawa et al., 1981). The Nordstrom¨ group identified antisense RNA-mediated control in a low-copy- number plasmid called R1 (Stougaard et al., 1981). In subsequent years, it became apparent that the negative control circuits that operate to “count” plasmids in a cell, and that correct deviations from the default copy number either use so- called iteron control (not covered here, but see Chattoraj, 2000) or antisense RNAs as the principal regulatory elements. Since much of our current under- standing of antisense RNA functions and mechanisms is derived from several well-characterized plasmids, a substantial part of this review will be devoted to these systems. 364 Table12.1. Prokaryotic Antisense RNAs, Occurrence, Mechanisms, and Biological Roles Antisense RNAa Biological function Target(s) Mechanism Comments Referencesb Plasmids IncFII Replication control repA mRNA Translation inhibition Light and Molin, 1982; relatives—CopA Womble et al., 1985 RNA IncB and IncIα Replication control repZ mRNA Translation inhibition, Hama et al., 1990; relatives—RNAI inhibition of activator Praszkier et al., 1991 pseudoknot ColE1 Replication control RNAII Primer maturation Rom protein Tomizawa et al., 1981 relatives—RNAI (preprimer) involvement pT181 and pIP501 Replication control repC mRNA Transcription attenuation Unusually stable Brantl et al., 1993; relatives—RNAI antisense RNA Novick et al., 1989 ColE2 relatives—RNAI Replication control rep mRNA Translation inhibition Takechi et al., 1994 pLS1 Replication control cop-rep mRNA Translation inhibition del Solar and Espinosa, 1992 R1162—ct RNA Replication control repI mRNA Translation inhibition Kim and Meyer, 1986 pVT736-1—Cop RNA Replication control rep mRNA Translation inhibition (?) Galli and Leblanc, 1995 R1 and relatives—Sok Postsegregational mok/hok mRNA Translation inhibition Activity in absence Gerdes et al., 1997 RNA killing of gene locus pAD1—RNAII Postsegregational RNAI Translation inhibition First case in a Greenfield et al., 2000 killing Gram-positive host IncFI/FII relatives—FinP Control of traJ mRNA Translation inhibition Antisense RNA Frost et al., 1989; RNA conjugation stabilized by FinO Koraimann et al., 1991 protein Transposons IS10—RNA-OUT Transposition RNA-IN Translation inhibition Ma and Simons, 1990 (tnp mRNA) IS30—RNA-C Transposition tnp mRNA) Inhibition of translation Arini et al., 1997 elongation (?) Bacteriophages λ—OOP RNA Lysis/lysogeny switch cII mRNA mRNA stability Krinke and Wulff, 1990 P1/P4—c4/cI RNA Lysis/lysogeny switch icd-ant mRNA Transcription Citron and Schuster, 1990; termination Sabbattini et al., 1995 P22—Sar RNA Lysis/lysogeny switch arc-ant mRNA Translation inhibition Liao et al., 1987 P22—Sas RNA Superinfection sieB-esc mRNA Switch of translation Ranade and Poteete, 1993 override start sites H-Tant Lysis/lysogeny switch T1 RNA RNA processing Stolt and Zillig, 1993 Bacterial E. coli MicF Stress responses, ompF mRNA Translation inhibition, Aiba et al., 1987 osmoregulation mRNA stability (?) E. coli OxyS Oxidative stress fhlA mRNA Translation inhibition Regulator of ∼40 Altuvia et al., 1998; rpoS mRNA Translation inhibition/ genes, acts as an Zhang et al., 1998 sequestration of Hfq antimutator E. coli DrsA Stress responses rpoS mRNA Translation activation Two (or more) targets, Sledjeski et al., 1996 hns mRNA Translation inhibition/ one activated mRNA stability E. coli DicF Cell division ftsZ mRNA Translation inhibition Encoded by prophage Tetart and Bouche, 1992 E. coli Sof Cell killing gef mRNA Translation inhibition Chromosomal hok/sok Poulsen et al., 1991 homolog S. aureus RNAIII Virulence hla mRNA Activation of translation Activator antisense Morfeldt et al., 1995; RNA Novick et al., 1993 V. anguillarum RNAα Iron transport fatA/fatB mRNA stability Salinas et al., 1993 regulation mRNAs B. subtilis tRNAs aminoacyl-tRNA aa-tRNA Transcription Grundy and Henkin, 1994 synthetase synthetase antitermination regulation mRNAs C. acetobutylicum Nitrogen glnA mRNA Translation inhibition (?) Janssen et al., 1990
Details
-
File Typepdf
-
Upload Time-
-
Content LanguagesEnglish
-
Upload UserAnonymous/Not logged-in
-
File Pages39 Page
-
File Size-