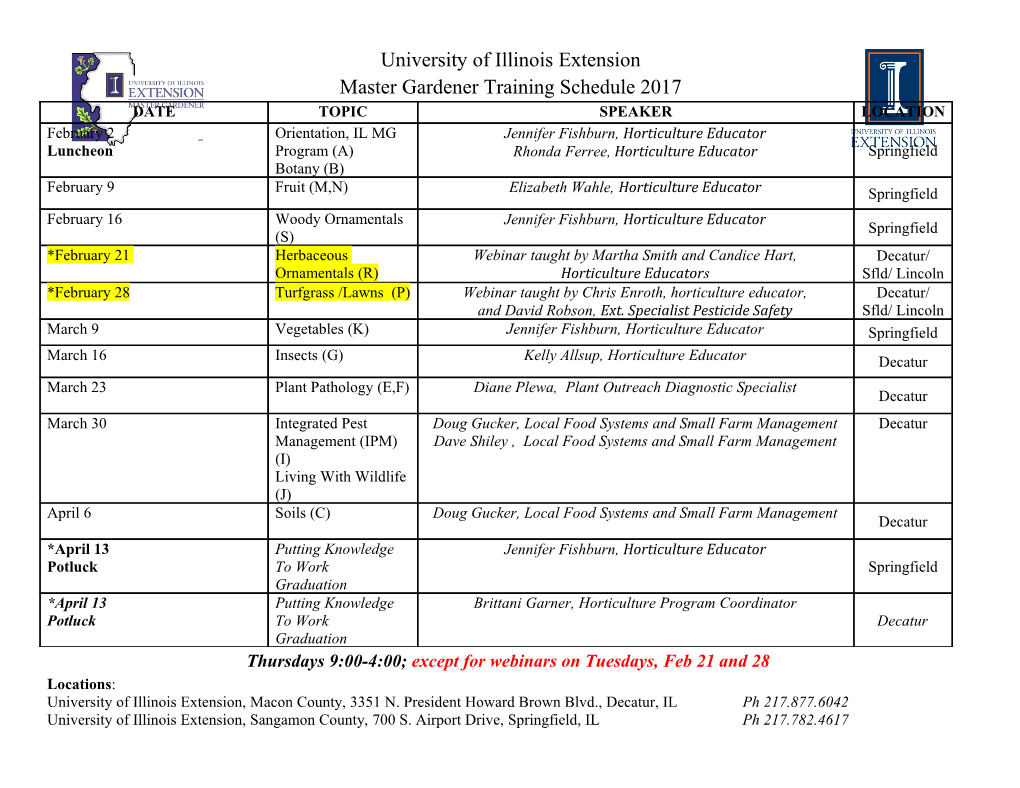
TNO-NITG – INFORMATION, December 2001 Building a 3D model of a gas field for geomechanical modelling The main objective of geomechanical modelling is to effectively predict surface and subsurface deformation and damage due to the exploitation of subsurface natural resources and/or the Research framework subsurface storage of energy residues (e.g., hazardous waste TNO-NITG, the geoscience institute and CO2). This objective is commonly achieved through of TNO, and TNO Building and two-dimensional (2D) stress analysis, as illustrated in the Construction Research have entered case study of a depleting gas reservoir presented in the previous into a close partnership for building integrated technological products issue of InFormation (May 2001). In many cases, however, the for geomechanical modelling to conventional 2D approach tends to oversimplify geological be applied to case studies. This structures and the importance of pre-existing tectonic stresses. institutional cooperation combines In this and the upcoming issue of InFormation, we will the cutting-edge geomechanical demonstrate that it is technically possible to incorporate into expertise of TNO Building and the geomechanical model both the full complexity of the Construction Research, which is particularly renowned for creating three-dimensional (3D) geological structure of a reservoir the world’s leading geomechanical and the possible variations in the tectonic stress field. code, DIANA (2000), with the cutting-edge expertise in integrated Workflow for integrated model the 3D geometry, while relying 3D geoscience modelling approaches geomechanical modelling on DIANA (DIANA, 2000) for the finite housed in TNO-NITG. We developed a workflow for inte- element (FE) geomechanical modelling. grated 3D geomechanical modelling Why 3D modelling? to accurately predict deformation. The modelling process comprises By adopting a 3D modelling approach, The workflow integrates the tools three logical steps (Figure 1): we can overcome most of the for geological modelling, fluid flow 1. Mapping and modelling, in which a shortcomings of the conventional modelling and stress analysis, structural model is built. 2D approach, which is unquestionably allowing efficient transfer of data 2. Characterisation, in which suited to modelling relatively simple between the shared earth models. property models are built by geological structures and pre-existing We used GOCAD (GOCAD, 2001) to assigning volumetric identifiers tectonic stresses. The pitfalls of the Reservoir 2D approach stem from the following: Mapping characterization Engineering applications - The model cross-section has to be Build framework Fluid flow modelling Geomechanical modelling perpendicular to the geological Structural Populate with Fluid modelling structure and, at the same time, IO model properties Geomechanic modelling the maximal principal stress and Feedback IO Property Existing module the minimal principal stress of the model Module to develop tectonic stress field must both lie Fluid flow & Build quality Model Upscale flow-stress mesh on the section, which is seldom the analysis IO Input/Output Geomechanical case. model - Complex stress regimes, such as Set boundary and loading Geomechanical a strike-slip regime, can not be conditions FE analysis modelled. Strain - The choice of material models is localization Deformation/ Downscale Micro fracturing generally restricted to those history tectonics Large models that are independent of displacements the intermediate principal stress (e.g., the Mohr-Coulomb model). Integrated visualization Figure 1. Workflow for integrated geomechanical modelling 5 TNO-NITG – INFORMATION, December 2001 Some of the geometric operations determines the density of triangles that might have to be carried out on in the surface patches. This, in turn, surfaces commonly involve filling the determines the density of the gaps in surfaces by interpolation, tetrahedron mesh. The resampled extending surfaces by extrapolation, keylines provide a starting point for clipping surfaces and finding surface generating resampled triangulated intersections. These geometric surfaces, which approximate the operations may alter the regular original surfaces of the structural model. The resulting surface patches marked by quality triangles define a boundary representation model that a. Gridded horizon and fault in the framework model is topologically and volumetrically consistent (Figure 2e). Such a model can be meshed successfully by a 3D (e.g., lithology) tetrahedral mesher, such as the one and properties available for pre-processing in (e.g., porosity) to DIANA. the volumes in the structural model. Case study 3. Engineering We will test the procedure analysis, which gives described above on a case b. Faulted horizon us predictions of study of a depleting gas surface and subsurface deformation, reservoir and show that it the assessment of which is the main is technically possible to objective of the geomechanical incorporate the complexity modelling. of the 3D geological structure of a reservoir into Method for structural a geomechanical model. modelling In order to create consistent 3D models of the subsurface geology c. Structural model in that will preserve the necessary GOCAD degree of geological complexity and be readily convertible into partitioning of the surfaces unstructured FE meshes, we due to incorporation of the developed the following procedure. intersection curves. Many The main steps of this procedure are additional vertices, and schematically presented in Figure 2. therefore triangles, are introduced into the surface The inputs for the modelling are along each intersection depth surfaces of differentiated curve. As a result, some d. Building the key-lines and key geological units and fault surfaces triangles and tetrahedrons in surfaces mapped in a mapping and contouring the FE mesh will have elongated package (Figure 2a). These surfaces and distorted shapes which are not are usually available as gridded acceptable for producing a good, surfaces and first have to be converted quality mesh. This problem can to triangulated surfaces (Figure 2b). be overcome by resampling all The surfaces also require some borderline segments that bound additional editing, for which GOCAD model surfaces at regular intervals provides a versatile set of tools, (Figure 2d). in order to develop a consistent e. Boundary representation model 3D structural model of the site of The interval length can be used to Figure 2. Example of a faulted structural model in the interest (Figure 2c). control the density of vertices along workflow. The data for modelling are taken from the Digital the line segments, which, in turn, Atlas of the Deep Subsurface of the Netherlands (TNO-NITG) 6 TNO-NITG – INFORMATION, December 2001 Geological setting The gas field under study is situated in the north-eastern region of the Base Tertiary faults Netherlands. This region is well known in the gas industry because of its large Permian Rotliegend fields, including the giant Slochteren Field on the Groningen High. In addition to these large fields, smaller occurrences Roswinkel Field can be found in younger deposits, (Triassic) such as in the gas field under study. The reservoir of this gas field consists of clastic sediments from the Lower Germanic Trias Group, found at a depth of around 2400 m. These clastic fluvio-lacustrine sediments were deposited in the intracratonic Zechstein salt Cretaceous Niedersaksian Basin which spreads subcrop out into Germany (Figure 3). Figure 4. Geological model of the Roswinkel gas field. This model is taken Lauwerszee Groningen High from the publicly available 3D Digital Atlas of the Deep Subsurface of the Trough Netherlands and visualised in a 3D Java viewer, developed by TNO-NITG H a n t u m compression that culminated in the F a u lt Ems Low Late Cretaceous. The compressional zo n e deformation resulted in inversion of the Late Jurassic faults and generated WSW-ENE folds truncated by the Friesland H o Roswinkel gas field Base Tertiary unconformity (Figure 4). l Platform s l o o The Base Tertiary structure shows t F a u NW-SE to NS trending grabens in the l t z o n area that are in close agreement with e the largest present-day horizontal Lower Saxony Basin stresses oriented NW-SE. Figure 3. Location of the gas Seismic events started to occur after field under study (green area). Dalfsen Tubbergen 12 years of production. The magni- High High The reservoir is positioned in an tudes recorded ranged from 1 to 3.5 anticlinal structure, delineated 0 20 km in Figure 4 on the Richter scale. All of the epicentres are located above the crest The reservoir structure is formed by subsequently, the deposition of the of the field. Depths of hypocentres an anticlinal structure above the Triassic sediments. In the Jurassic have been indicated at or above Emmen Salt dome. Figure 4 shows period, more differentiated subsidence reservoir depth, with an uncertainty the geological structure in the occurred through extensional WSW- of ±500 m in the vertical direction. vicinity of the reservoir. The basin ENE trending faults soling into the evolution of the Niedersaksian Basin Permian evaporites. The extensional Geomechanical model is marked by various phases of faulting, which was most active The structural model and the tectonism, during which halokinetic during the Late Kimmeridgian boundary representation model of flow played an important role. In (ca. 150 Ma), caused halokinesis of the gas field under study, constructed the
Details
-
File Typepdf
-
Upload Time-
-
Content LanguagesEnglish
-
Upload UserAnonymous/Not logged-in
-
File Pages4 Page
-
File Size-