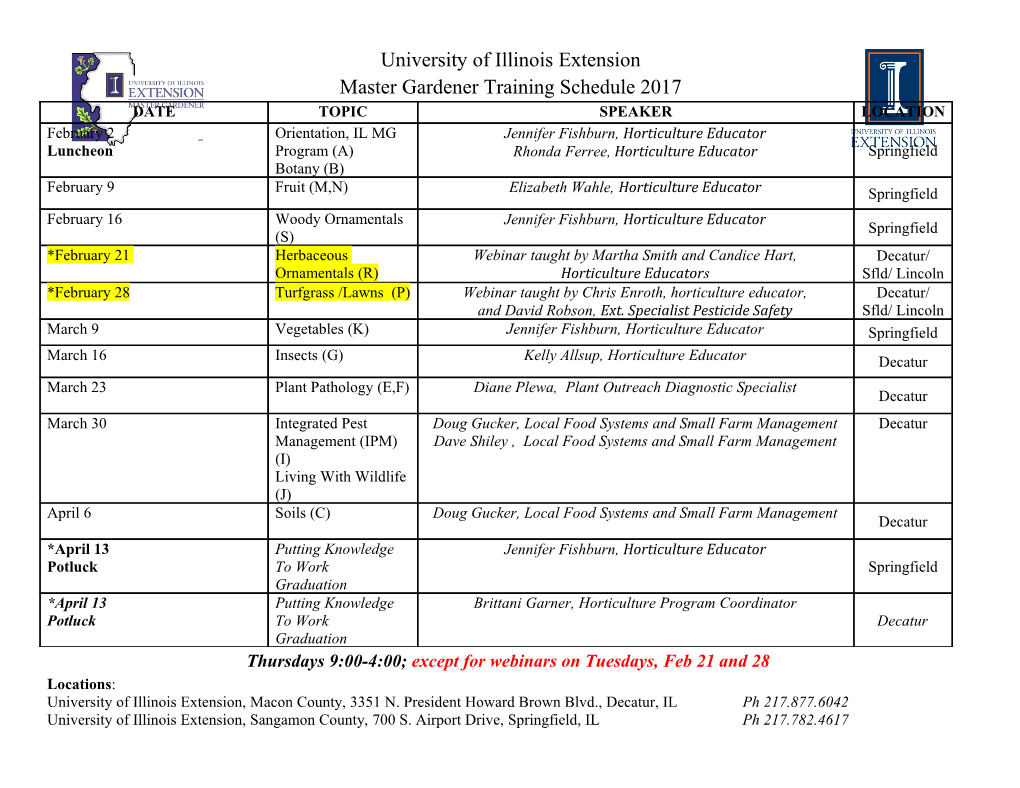
Ligand “noninnocence” in coordination complexes vs. kinetic, mechanistic, and selectivity issues in electrochemical catalysis Cyrille Costentina,1,2, Jean-Michel Savéanta,1, and Cédric Tardb,1 aUniversité Paris Diderot, Sorbonne Paris Cité, Laboratoire d’Electrochimie Moléculaire, Unité Mixte de Recherche Université, CNRS 7591, 75205 Paris Cedex 13, France; and bLCM, CNRS, Ecole Polytechnique, Université Paris-Saclay, 91128 Palaiseau Cedex, France Contributed by Jean-Michel Savéant, July 13, 2018 (sent for review June 21, 2018; reviewed by Marc Fontecave and Clifford P. Kubiak) The world of coordination complexes is currently stimulated by as to where the charge is located but is merely taking care of the the quest for efficient catalysts for the electrochemical reactions bookkeeping of the number of electrons globally exchanged. In underlying modern energy and environmental challenges. Even in the framework of molecular catalysis of electrochemical reac- the case of a multielectron−multistep process, catalysis starts with tions, designing selective efficient catalysts and benchmarking a uptake or removal of one electron from the resting state of the catalyst requires kinetic and mechanistic insights. Relying on catalyst. If this first step is an outer-sphere electron transfer (trig- electronic distribution in the catalyst resting state to predict re- gering a “redox catalysis” process), the electron distribution over activity involving the ligand or the metal may be misleading, as the metal and the ligand is of minor importance. This is no longer shown later on. the case with “chemical catalysis,” in which the active catalyst There is actually no obvious relationship between ligand non- reacts with the substrate in an inner-sphere manner, often involv- innocence and catalytic efficiency that could be used, per se, to ing the transient formation of a catalyst−substrate adduct. The help benchmark catalysts and design more efficient ones. Pursuing fact that, in most cases, the ligand is “noninnocent,” in the sense these goals requires gathering kinetic or transient information that the electron density and charge gained (or removed) from the through application of molecular electrochemistry concepts and resting state of the catalyst are shared between the metal and the techniques. Strategies in this area are enlightened by delineation ligand, has become common-place knowledge over the last half- of two types of catalyzed electrochemical processes (Fig. 1) (4). “ ” century. Insistent focus on a large degree of noninnocence of the In redox catalysis, the active form of the catalyst acts as a mere outer-sphere single-electron transfer. In the other—“chemical ligand in the resting state of the catalyst, even robustly validated ”— by spectroscopic techniques, may lead to undermining the essen- catalysis a catalytic intermediate is formed by an inner-sphere tial role of the metal when such essential issues as kinetics, mech- reaction between the electron transfer-generated active form of the catalyst and the substrate, opening a reaction sequence that anisms, and product selectivity are dealt with. These points are regenerates the starting form of the catalyst and is kinetically more general in scope, but their discussion is eased by adequately docu- favorable than a mere outer-sphere electron transfer. This Sabatier mented examples. This is the case for reactions involving metal- intermediate (5) may be simply an adduct formed by addition of the loporphyrins as well as vitamin B12 derivatives and similar cobalt substrate to the active form of the catalyst (QA in Fig. 1) or another complexes for which a wealth of experimental data is available. type of catalytic intermediate formed with a lower barrier than that of an outer-sphere electron transfer. The identification and the electrochemical reactions | catalysis | contemporary energy challenges | structural characterization of trapped intermediates are ways of ligand/metal noninnocence | cyclic voltammetry unraveling mechanisms, with the possible drawback that the mechanism and kinetics of the catalytic reaction under normal atalysis of electrochemical reactions by low-valent (for re- Cductions; high-valent for oxidations) coordination metal Significance complexes is a topic that has recently garnered boosted atten- tion triggered by modern energy and environmental chal- Coordination complexes are constantly sought as catalysts in lenges. Considering, for example, the case of reductive processes, the transformation of small molecules involved in contempo- the question may arise of whether the electron introduced in the rary energy and environmental challenges. Insistent emphasis starting complex to produce the catalytic species will “sit” on the on the well-established notion of ligand “noninnocence” may metal or on the ligand (or, symmetrically, where is the hole lo- blur the essential role of the metal center when kinetic, cated in case of oxidations), therefore hopefully orienting the mechanistic, and selectivity issues are targeted rather than the follow-up chemistry toward catalysis. This makes us inescapably detailed electronic structure of the starting catalyst molecule. enter the world of “noninnocent ligands,” a terminology that has With the help of modern concepts and techniques of molecular flourished after it was realized that, in redox reactions, both the electrochemistry, several examples are described in which ex- metal and the ligand can host the incoming electron in reduc- tremely efficient catalysis takes place at the metal center de- tions (or holes in oxidations). An excellent retrospective (1) spite an a priori discouraging large delocalization of charge and traces back the origin of this picturesque expression of the rather electron density on the noninnocent ligand. straightforward notion that the metal and ligand orbitals mix, forming global molecular orbitals. The repetitive recent use of Author contributions: C.C., J.-M.S., and C.T. designed research, performed research, and this terminology (2, 3) is thus surprising, noting that the weight of wrote the paper. the ligand contribution in these global molecular orbitals has Reviewers: M.F., Collège de France; and C.P.K., University of California, San Diego. indeed no reason to be negligible, the more so if the ligand has The authors declare no conflict of interest. intrinsic redox properties or, simply, electronic withdrawing/do- Published under the PNAS license. nating ability that render it noninnocent. The same is re- 1To whom correspondence may be addressed. Email: cyrille.costentin@univ-paris-diderot. spectively true for the metal. Conventional designation of the fr, [email protected], or [email protected]. oxidation state of a metallic complex by the formal oxidation 2Present address: Department of Chemistry and Chemical Biology, Harvard University, state of metal, the ligand being viewed as perfectly “innocent” Cambridge, MA 02138. and the metal perfectly “guilty,” has obviously no realistic scope Published online August 24, 2018. 9104–9109 | PNAS | September 11, 2018 | vol. 115 | no. 37 www.pnas.org/cgi/doi/10.1073/pnas.1810255115 Downloaded by guest on October 1, 2021 electrode solution direct Catalytic and Noncatalytic Reactions of Electrogenerated - A e electrochemical Low-Valent Metalloporphyrins, Vitamin B12s, and Similar reaction potential redox catalysis energy products (no selectivity) Cobalt Complexes B “I”/“0” products (no selectivity) The Fe porphyrin couple is involved in several catalytic e- P B redox catalyzed two-step chemical catalysis processes, notably catalysis of the CO2-to-CO electrochemical electrochemical QA reaction conversion, where the systematic analysis of ligand substitution A Q Q + A has led to the design of the most efficient catalysts of this re- B Product (selectivity) P + B action today (7–9). As detailed later on, the Fe0 resonance form e- P chemically catalyzed QA (or CI) is considered, among the mesomeric forms, as the best repre- electrochemical reaction QA no catalysis sentation of the active catalyst at the start of the catalytic loop, Q Â Ã Â Ã Â Ã 0,2− I,− II A reaction coordinate Fe ðPÞ ↔ Fe ðP · −Þ ↔ Fe ðP :; 2 − Þ . Fig. 1. Contrasting redox and chemical catalysis of an electrochemical re- action (in the case of a reduction). A, substrate; B, products; P/Q, the catalyst From the very first times the catalytic or stoichiometric reactivity couple; QA, the Sabatier adduct or intermediate. of doubly reduced FeII porphyrin were investigated, until very recently, various spectroscopic techniques have indicated that the FeII resonance form appears as largely predominant over the operating conditions would not be the simple extension of what they 0 Fe form (10–21). Taking as an example the reduction of CO2 to are under intermediate trapping conditions. A particularly effective CO, the starting molecular reductant would be the FeII reso- way of investigating and operating electron transfer-triggered ca- nance form of the doubly reduced FeII porphyrin and the final talysis is offered by molecular electrochemistry. Control of the product FeIICO. From this perspective, the metal oxidation electrode potential provides a continuous variation of the concen- number seems to be invariant over the course of the reaction, tration of the active catalyst at the electrode, while the current af- and hence the ligand is not only noninnocent but also “redox fords straightforward access to the catalytic reaction kinetics. An active.” However, in this example as
Details
-
File Typepdf
-
Upload Time-
-
Content LanguagesEnglish
-
Upload UserAnonymous/Not logged-in
-
File Pages6 Page
-
File Size-