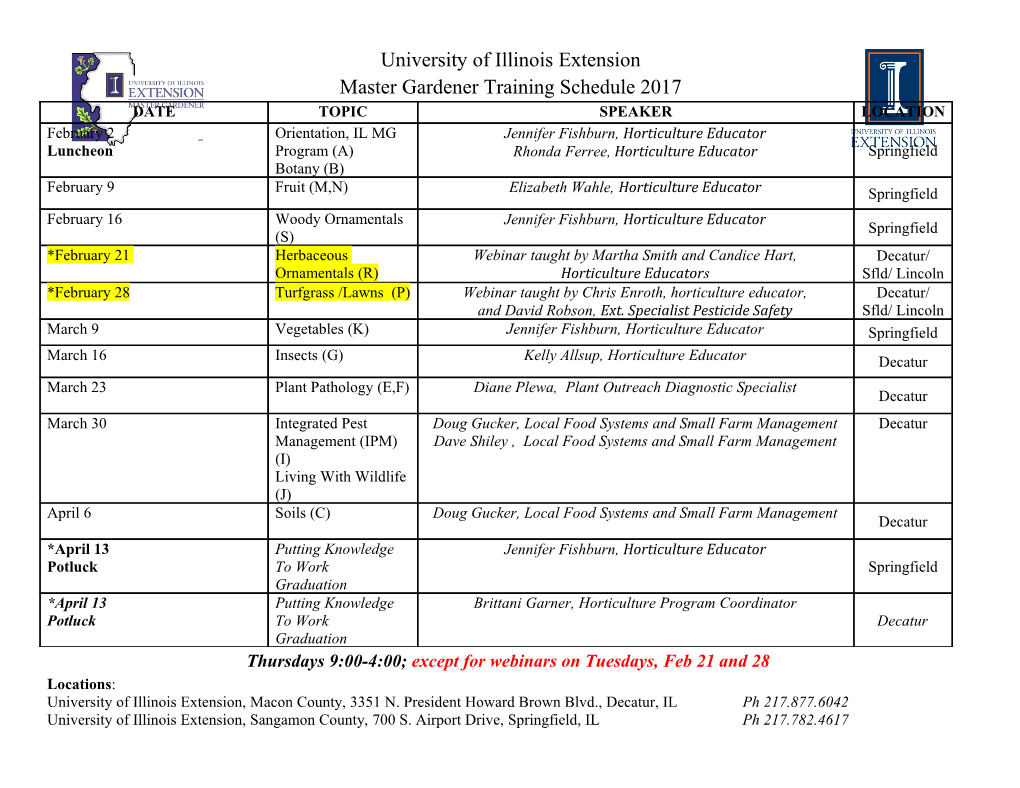
PNNL-21515 Prepared for the U.S. Department of Energy under Contract DE-AC05-76RL01830 Prognostics and Health Management in Nuclear Power Plants: A Review of Technologies and Applications JB Coble P Ramuhalli LJ Bond JW Hines BR Upadhyaya July 2012 PNNL-21515 Prognostics and Health Management in Nuclear Power Plants: A Review of Technologies and Applications JB Coble P Ramuhalli LJ Bond(a) JW Hines(b) BR Upadhyaya(b) July 2012 Prepared for the U.S. Department of Energy under Contract DE-AC05-76RL01830 Pacific Northwest National Laboratory Richland, Washington 99352 ___________________ (a) Formerly Lab Fellow at PNNL Currently Director of CNDE at Iowa State University Ames, Iowa 50011 (b) The University of Tennessee Knoxville, Tennessee 37996 Abstract Recent years have seen a resurgence of nuclear power worldwide, with interest in extending the operating life of the approximately 436 reactors currently in service (as of March, 2012), 61 new reactors being constructed, and as many as 162 under consideration. Renewed worldwide interest in nuclear power has been somewhat tempered by the March 2011 incident at Fukushima Dai-ichi in Japan. However, nuclear power is still considered a key element in meeting future worldwide sustainable energy, energy security, and emissions goals. Currently, three separate thrusts to safe and economical nuclear power development for energy security are being pursued in the United States: (i) longer term operation for the legacy fleet, from 40–60 and possibly 60–80 years; (ii) near-term new nuclear plants with a 60-year design life; and (iii) small modular reactors, which are expected to employ light water reactor technology at least in the medium term. Within these activities, attention is turning to enhanced methods for plant component and structural health management. The operating U.S. fleet includes 104 light water reactors. In addition, there are now (as of May 2012) four new nuclear power plants (AP-1000 plants) under construction in the United States, and two delayed plants are being completed by the Tennessee Valley Authority. There is also interest in the United States in small modular reactors (SMRs), which could be easier to match to existing grid infrastructure and which could replace aging coal fired plants. The current low price for natural gas presents a challenge to the economics of nuclear power, at least in the short term; however, some recent studies have demonstrated that nuclear generation will be competitive in the longer term (at least in some markets) when anticipated escalation in gas prices and the cost of building, operating, and maintaining gas-fired plants are considered over those same time periods. This report reviews the current state of the art of prognostics and health management (PHM) for nuclear power systems and related technology currently applied in field or under development in other technological application areas, as well as key research needs and technical gaps for increased use of PHM in nuclear power systems. The historical approach to monitoring and maintenance in nuclear power plants (NPPs), including the Maintenance Rule for active components and Aging Management Plans for passive components, are reviewed. An outline is given for the technical and economic challenges that make PHM attractive for both legacy plants through Light Water Reactor Sustainability (LWRS) and new plant designs. There is a general introduction to PHM systems for monitoring, fault detection and diagnostics, and prognostics in other, non-nuclear fields. The state of the art for health monitoring in nuclear power systems is reviewed. A discussion of related technologies that support the application of PHM systems in NPPs, including digital instrumentation and control systems, wired and wireless sensor technology, and PHM software architectures is provided. Appropriate codes and standards for PHM are discussed, along with a description of the ongoing work in developing additional necessary standards. Finally, an outline of key research needs and opportunities that must be addressed in order to support the application of PHM in legacy and new NPPs is presented. iii Summary Recent years have seen a resurgence of nuclear power worldwide, with interest in extending the operating life of the approximately 436 reactors currently in service (as of March 2012), 61 new reactors being constructed, and as many as 162 under consideration. While the renewed interest in nuclear power has been somewhat tempered by the March 2011 incident at Fukushima Dai-ichi in Japan, nuclear power is still considered key to meeting future worldwide sustainable energy, energy security, and emissions goals. Currently, three separate thrusts to safe and economical nuclear power development for energy security are being pursued in the United States. The first thrust is focused on life extension of operating light-water reactors (LWRs), referred to as long-term operation (LTO). In the United States, 71 reactors have been approved for license extension from 40 years to 60 years of operation, and 9 plants have already entered LTO (in some cases, with power uprates). Similar trends are being reported worldwide. Interest is now turning to a second license extension to enable operation from 60–80 years, so-called extended LTO or life-beyond-60 (LB60). The question of safely and economically operating some of these plants beyond 60 years is now being considered in anticipation of this second round of license extensions. The existing fleet is considered to be too valuable to decommission, even if $1B per plant is needed for refurbishment to give another 20 years of operation. Through power uprates of existing plants, U.S electric companies have added the equivalent of five or six new reactors in the past 30 years by upgrading legacy plants in order to produce more power from the same plant (Carter 2006). The second pathway focuses on new LWR reactor designs. In the United States, the Westinghouse AP-1000 has emerged as the primary Generation III+ design, and four plants are currently under construction. The AP-1000 has enhanced safety features and a 60-year design life. Similar features are being included in other Generation III+ designs, including the ABWR, APWR, EPR, EBR, and Advanced CANDU Reactor (ACR), many of which are being built worldwide. The third thrust is in the area of advanced reactor designs that rely on passive safety systems for decay heat removal; in the near-term, there is a focus on light water reactor designs and a particular focus on so-called small modular reactors (SMRs). Over the years, operational experience has shown that greater situational awareness of the state of safety-critical nuclear plant systems, structures, and components is necessary, particularly as they age over time due to exposure to harsh service conditions. While replacement of a subset of components is possible, it may be economically prohibitive to replace several of the larger components, including the reactor pressure vessel and primary piping. Thus, detection, management, and mitigation of aging-related degradation in these critical components become important to maintain safety margins. In this context, the technical challenges related to detecting, characterizing, monitoring, and managing materials degradation need to be identified and addressed. These challenges are not unique to the operating fleet of LWRs, as the next generation of nuclear power reactors is expected to have similar needs in terms of managing and mitigating degradation; therefore, it is likely that any technology developed for detecting and characterizing degradation will have applications beyond the current fleet. Additionally, some next- generation reactor designs have potentially increased monitoring needs due to extended fuel cycles, reduced access to critical components, and remote siting with reduced maintenance staff. v The key technology developments necessary for detecting and managing degradation in reactor components are: (1) nondestructive measurement methods and analyses to detect degradation and anomalies, (2) algorithms to characterize and monitor the degradation state of the component, and (3) algorithms that use the degradation state information to determine remaining useful life (RUL) and probability of failure (POF) of the component. The POF information may then be used in a probabilistic risk assessment (PRA) model to assess the risk significance of the degradation and the corresponding reduced safety margin. Together, these technologies constitute prognostics and health management (PHM) systems. Ideally, degradation detection should occur early in the degradation development lifecycle, to enable the application of appropriate mitigation or repair actions, thereby maintaining the necessary safety margins. Appropriate PHM systems therefore can potentially preclude serious consequences due to aging-related faults. This report reviews the current state of the art of prognostics and health management for nuclear power systems and related technology currently applied in field or under development in other technological application areas. The nuclear power industry worldwide poses a unique challenge for application of health management systems. NPPs worldwide are closely regulated by national and international licensing bodies, and application of PHM systems which may impact safety-critical systems (and some non-safety– related systems) will require a clear licensing basis outlining appropriate algorithms, architectures, and applications.
Details
-
File Typepdf
-
Upload Time-
-
Content LanguagesEnglish
-
Upload UserAnonymous/Not logged-in
-
File Pages124 Page
-
File Size-