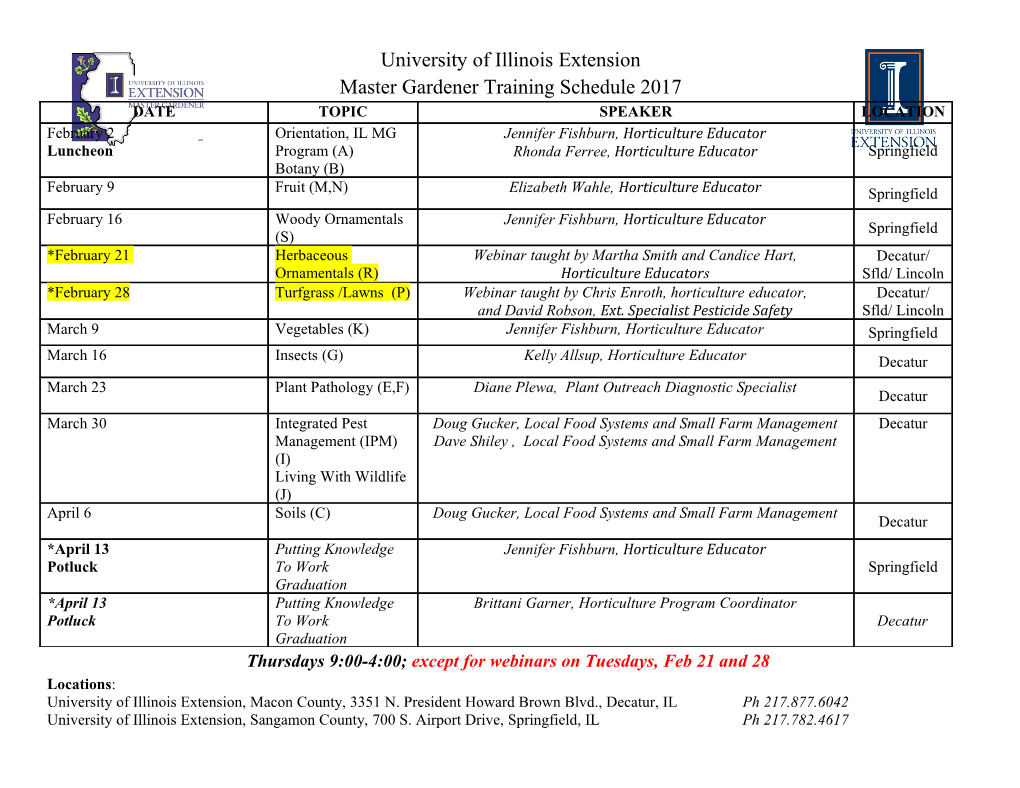
Creation of Ultracold RbCs Ground-State Molecules DISSERTATION by Markus Debatin submitted to the Faculty of Mathematics, Computer Science and Physics of the University of Innsbruck in partial fulfillment of the requirements for the degree of doctor of science advisor: Univ.Prof. Dr. Hanns-Christoph N¨agerl, Institute of Experimental Physics, University of Innsbruck Innsbruck, July 2013 Abstract Control of external and internal degrees of freedom at the level of single quantum states is essential for a series of molecular physics experiments. Heteronuclear dimers feature a large electric dipole moment, which makes them particular interesting candidates for experiments with strongly interacting quantum gases. However efficient cooling schemes such as laser cooling are difficult to realize for molecules. Therefore we create ultracold molecules from already cooled ultracold gases. This this work presents a series of experiments that has been carried out in order to create the molecules in their lowest internal quantum state. Techniques to create ultracold atoms are readily available. However, the creation of stable mixtures is still a challenge since inter-species scattering plays a role in addition to intra-species scattering physics. This requires us to cool two clouds of 87Rb and 133Cs separately and merge the clouds afterwards. After overlapping the BECs, we produce weakly bound RbCs molecules using the Feshbach-association technique. We transfer the molecules from the weakly bound state to the lowest vibrational and rotational level of the X1Σ+ electronic ground-state potential. For the transfer the initial and the final state are linked with lasers to an intermediate electronically excited state. The transfer is achieved by the Stimulated Raman Adiabatic Passage (STIRAP) technique. In order to identify a suitable route for STIRAP a variety of electronically excited molecular levels is investigated by high resolution spectroscopy. Two-photon spectroscopy is used in order to determine the binding energy of the lowest ro-vibrational level of the 1 + X −1 X Σ ground state to be D0 = 3811.5755(16) cm . The vibrational level 29 of the 3 b Π1 electronic excited potential is determined to feature suitable couplings to both the initial and the final state and the molecules are transfered to the ground state with an efficiency of 89%. In order to determine the hyperfine level of the molecular ground state, the hyperfine splitting is measured and STIRAP transfer to a different vibrational level is carried out. It is found that for RbCs the Feshbach molecules can be directly transferred to the lowest hyperfine level of the ro-vibrational ground state. Zusammenfassung Diese Doktorarbeit umfasst experimentelle Studien zur Erzeugung ultrakalter dipolarer RbCs Grundzustandsmolek¨ule. F¨urdipolare Quantengase wird aufgrund der langreich- weitigen Dipol-Dipol Wechselwirkung eine Vielfalt neuartiger Quantenzust¨andein op- tischen Gittern vorhergesagt. F¨urden experimentellen Nachweis vieler dieser Quanten- zust¨andeist die Erzeugung eines Quantengases aus stark wechelwirkenden Dipolen es- sentiell. Heteronukleare Dimere weisen ein ausgepr¨agteselektrisches Dipolmoment auf, welches es erm¨oglicht, Systeme mit starker Dipol-Dipol Wechselwirkung zu realisieren. Die derzeit einzige nachgewiesene M¨oglichkeit, ein molekulares Quantengas zu erzeu- gen, ist die Feshbach-Assoziation mit anschließendem Grundzustandstransfer durch einen stimulierten adiabatischen Raman-Ubergang¨ (STIRAP). Durch die Feshbachi-Assoziation werden zun¨achst freie Atome zu schwach gebundenen Molek¨ulen(Feshbach Molek¨ulen) verbunden, welche sich in einem hoch angeregten Vibrationszustand befinden. Der da- rauf folgende STIRAP transferiert die Mole¨ulevom angeregten Zustand in den moleku- laren Grundzustand. Der Transfer in den Grundzustand ist notwendig, um inelastische Streuprozesse zu verhindern. Zudem ist das Dipolmoment heternonuklearer Molek¨ulein niedrigen Vibrationszust¨andenbesonders stark ausgepr¨agt. Auf diese Weise wurde bereits erfolgreich ein Quantengas aus fermionischem KRb erzeugt. Im Rahmen unserer experimentellen Studien ist es gelungen, bosonische RbCs Molek¨uledurch Feshbach-Assoziation ultrakalter Rb und Cs Quantengase zu erzeugen und mittels STIRAP in ihren Grundzustand zu transferieren. Neben einem bosonis- chen Charakter und einem gr¨oßerenDipolmoment unterscheidet sich RbCs von KRb hinsichtlich der chemischen Stabilit¨at.KRb kann bei einer Kollision zweier Molek¨ulezu K2+Rb2 reagieren. F¨urRbCs sind solche Reaktionen endotherm und in ultrakalten Gasen aufgrund der niedrigen kinetischen Energie nicht m¨oglich. Daher ist RbCs im Grundzus- tand stabil. Dies kann einen entscheidenden Vorteil f¨urviele Experimente darstellen. Zur Erzeugung ultrakalter RbCs Molek¨ulewerden zun¨achst zwei separate ultrakalte Atomwolken aus Rb und Cs generiert. Aus den gemischtem Atomwolken werden hernach schwach gebundene Feshbach-Molek¨ulenassoziiert. Um die Molek¨ulemittels STIRAP in den Grundzustand zu transferieren, ist es n¨otig,geeignete elektronisch angeregte Vibra- tionsniveaus zu identifizieren. Daher wird eine Reihe von Vibrationsniveaus hinsichtlich ihrer Eignung f¨urSTIRAP spektroskopisch untersucht. Letzteres umfasst auch die Bes- timmung ihrer Energie sowie ihrer Kopplung an die Feshbach-Molek¨uleund den Grundzu- stand. Im Rahmen der spektroskopischen Untersuchungen wird die Bindungsenergie des 1 + X −1 niedrigsten Vibrationsniveaus des X Σ Grundzustandes zu D0 = 3811.5755(16) cm bestimmt. Die hochaufl¨osendeSpektroskopie erlaubt es ferner, einzelne Hyperfeinzust¨ande zu detektieren. Ausgehend von den gewonnenen Daten wird ein geeignetes angeregtes Niveau aus- gew¨ahlt,und die Molek¨ulewerden mit einer Effizienz von 89% in den Grundzustand transferiert. Dabei gelingt es, die Mole¨uklein das niedrigste Hyperfeinniveau des moleku- laren Grundzustandes zu transferieren. Die Arbeit zeigt im Detail, daß ein effizienter Grundzustandstransfer selbst bei geringen Kopplungsst¨arken m¨oglich ist und analysiert Faktoren, die die Effizienz limitieren. Contents 1 Introduction1 I Physics and creation of ultracold dipolar molecules7 2 Dipolar quantum gases9 2.1 Dipole-dipole interactions........................... 11 2.2 Scattering properties.............................. 14 2.3 Dipolar molecules in an electric field..................... 15 2.4 Optical lattices................................. 17 2.5 Dipolar molecules in reduced dimensions................... 20 2.6 Possible applications of dipolar quantum gases................ 21 3 Ultracold molecules 23 3.1 Cooling methods................................ 24 3.2 Creation of mixtures.............................. 28 3.2.1 Cooling of atoms............................ 29 3.2.2 Atoms in traps............................. 30 3.2.3 Collisions and losses.......................... 33 3.2.4 Feshbach resonances.......................... 36 3.3 Association of molecules............................ 39 3.3.1 Feshbach association.......................... 39 3.3.2 Radio-frequency association...................... 40 3.3.3 Photoassociation............................ 41 3.3.4 Enhanced association from pairs of atoms.............. 41 3.4 Quantum numbers and properties of ultracold molecules.......... 43 3.4.1 Reactive collisions............................ 45 II The RbCs experiment 47 4 Experimental setup 49 4.1 Setup for creation of an ultracold Rb-Cs mixture.............. 49 4.1.1 Rb and Cs atomic properties...................... 49 4.1.2 Creation of cold atomic gases..................... 51 4.1.3 Magnetic and electric fields...................... 51 4.1.4 Simultaneous condensation of Rb and Cs............... 53 v Contents 4.2 Laser setup for spectroscopy and ground-state transfer........... 55 4.2.1 Piezo cavity setup............................ 58 4.2.2 PDH error signals............................ 60 4.2.3 High finesse cavity........................... 61 4.2.4 Frequency tuning............................ 63 4.2.5 Ambient magnetic field noise...................... 64 5 RbCs Feshbach molecules 67 5.1 Feshbach structure of RbCs.......................... 67 5.2 Creation of Feshbach molecules........................ 71 5.2.1 Association into the | − 6(2, 4)d(2, 2)i state.............. 71 5.2.2 Association into the | − 6(2, 4)d(2, 4)i state.............. 73 5.2.3 Lifetime of Feshbach molecules.................... 73 5.2.4 Magnetic moment spectroscopy.................... 74 5.2.5 Characterization of Feshbach molecules................ 75 6 Spectroscopy 77 6.1 Molecular structure............................... 78 6.2 Excited state spectroscopy........................... 80 1 + 3 6.2.1 Spectroscopy of the A Σ − b Π0 potential............. 80 3 6.2.2 Spectroscopy of the b Π1 potential.................. 85 6.2.3 Summary of the excited states..................... 87 6.3 Two-photon dark-state resonance spectroscopy................ 90 6.3.1 Resonant spectroscopy......................... 92 6.3.2 Three-level systems with off-resonant coupling............ 93 6.3.3 Measurement of the decoherence.................... 95 6.3.4 Detection of two-photon resonance.................. 96 6.3.5 Observation of Autler-Townes splitting................ 98 6.3.6 Hyperfine structure measured by two-photon spectroscopy..... 99 7 Coherent ground-state transfer 105 7.1 Introduction to stimulated Raman adiabatic passage (STIRAP)...... 105 7.2 Transfer efficiency in the presence of noise.................. 107 7.2.1 Modelling noise............................. 107 7.2.2 STIRAP in the presence of
Details
-
File Typepdf
-
Upload Time-
-
Content LanguagesEnglish
-
Upload UserAnonymous/Not logged-in
-
File Pages161 Page
-
File Size-