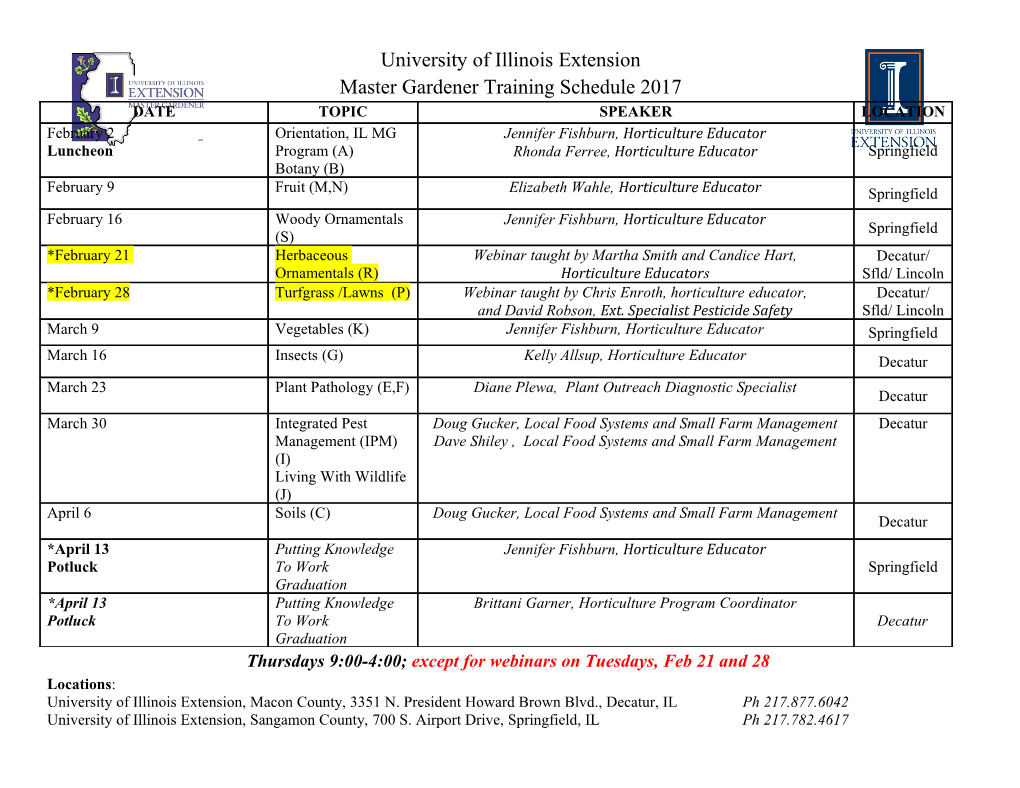
1 Graduate Texts Woo Young Lee Lecture Notes on Operator Theory Spring 2008 Seoul National University Seoul, Korea Copyright c 2008 by the Seoul National University 2 . Lecture Notes on Operator Theory Woo Young Lee 3 . Preface The present lectures are based on a graduate course delivered by the author at the Seoul National University, in the spring semester of 2008. In these lectures I attempt to set forth some of the recent developments that had taken place in Operator Theory. In particular, I focus on the Fredholm and Weyl theory, hyponormal and subnormal theory, weighted shift theory, Toeplitz theory, and the invariant subspace problem. Seoul February, 2008 The author 4 Contents 1 Fredholm Theory 7 1.1 Introduction ................................. 7 1.2 Preliminaries ................................ 8 1.3 Definitions and Examples ......................... 10 1.4 Operators with Closed Ranges ...................... 11 1.5 The Product of Fredholm Operators ................... 14 1.6 Perturbation Theorems .......................... 16 1.7 The Calkin Algebra ............................ 18 1.8 The Punctured Neighborhood Theorem . 23 1.9 The Riesz-Schauder (or Browder) Theory . 26 1.10 Essential Spectra .............................. 32 1.11 Spectral Mapping Theorems ........................ 33 1.12 The Continuity of Spectra ......................... 35 1.13 Comments and Problems ......................... 38 2 Weyl Theory 39 2.1 Introduction ................................. 39 2.2 Weyl’s Theorem .............................. 41 2.3 Spectral Mapping Theorem for the Weyl spectrum . 53 2.4 Perturbation Theorems .......................... 59 2.5 Comments and Problems ......................... 66 3 Hyponormal and Subnormal Theory 69 3.1 Hyponormal Operators ........................... 69 3.2 The Berger-Shaw Theorem ........................ 73 3.3 Subnormal Operators ........................... 78 3.4 p-Hyponormal Operators ......................... 89 3.5 Comments and Problems ......................... 93 4 Weighted Shifts 97 4.1 Berger’s theorem .............................. 97 4.2 k-Hyponormality .............................. 101 4.3 The Propagation .............................. 105 4.4 The Perturbations ............................. 112 5 CONTENTS 4.5 The Extensions ............................... 130 4.6 The Completion Problem . 139 4.7 Comments and Problems . 145 5 Toeplitz Theory 149 5.1 Preliminaries ................................ 149 5.1.1 Fourier Transform and Beurling’s Theorem . 149 5.1.2 Hardy Spaces ............................ 152 5.1.3 Toeplitz Operators . 154 5.2 Hyponormality of Toeplitz operators . 161 5.2.1 Cowen’s Theorem . 161 5.2.2 The Case of Trigonometric Polynomial Symbols . 165 5.2.3 The Case of Rational Symbols . 168 5.3 Subnormality of Toeplitz operators . 171 5.3.1 Halmos’s Problem 5 . 171 5.3.2 Weak Subnormality . 181 5.3.3 Gaps between k-Hyponormality and Subnormality . 187 5.4 Comments and Problems . 189 6 A Brief Survey on the Invariant Subspace Problem 191 6.1 A Brief History ............................... 191 6.2 Basic Facts ................................. 193 6.3 Quasitriangular operators . 194 6 Chapter 1 Fredholm Theory 1.1 Introduction If k(x; y) is a continuous complex-valued function on [a; b] £ [a; b] then K : C[a; b] ! C[a; b] defined by Z b (Kf)(x) = k(x; y)f(y)dy a is a compact operator. The classical Fredholm integral equations is Z b ¸f(x) ¡ k(x; y)f(y)dy = g(x); a · x · b; a where g 2 C[a; b], ¸ is a parameter and f is the unknown. Using I to be the identity operator on C[a; b], we can recast this equation into the form (¸I ¡ K)f = g. Thus we are naturally led to study of operators of the form T = ¸I ¡ K on any Banach space X. Riesz-Schauder theory concentrates attention on these operators of the form T = ¸I ¡ K, ¸ 6= 0, K compact. The Fredholm theory concentrates attention on operators called Fredholm operators, whose special cases are the operators ¸I ¡ K. After we develop the “Fredholm Theory”, we see the following result. Suppose k(x; y) 2 C[a; b] £ C[a; b] (or L2[a; b] £ L2[a; b]). The equation Z b ¸f(x) ¡ k(x; y)f(y)dy = g(x); ¸ 6= 0 (1.1) a has a unique solution in C[a; b] for each g 2 C[a; b] if and only if the homogeneous equation Z b ¸f(x) ¡ k(x; y)f(y)dy = 0; ¸ 6= 0 (1.2) a has only the trivial solution in C[a; b]. Except for a countable set of ¸, which has zero as the only possible limit point, equation (1.1) has a unique solution for every g 2 C[a; b]. For ¸ 6= 0, the equation (1.2) has at most a finite number of linear independent solutions. 7 CHAPTER 1. FREDHOLM THEORY 1.2 Preliminaries Let X and Y be complex Banach spaces. Write B(X; Y ) for the set of bounded linear operators from X to Y and abbreviate B(X; X) to B(X). If T 2 B(X) write ½(T ) for the resolvent set of T ; σ(T ) for the spectrum of T ; ¼0(T ) for the set of eigenvalues of T . We begin with: Definition 1.2.1. Let X be a normed space and let X¤ be the dual space of X. If Y is a subset of X, then Y ? = ff 2 X¤ : f(x) = 0 for all x 2 Y g = ff 2 X¤ : Y ½ f ¡1(0)g is called the annihilator of Y . If Z is a subset of X¤ then \ :?Z = fx 2 X : f(x) = 0 for all f 2 Zg = f ¡1(0) f2Z is called the back annihilator of Z. Even if Y and Z are not subspaces, and Y ? and :?Z are closed subspaces. Lemma 1.2.2. Let Y; Y 0 ½ X and Z; Z0 ½ X¤. Then (a) Y ½ :?(Y ?), Z ½ (?Z)?; (b) Y ½ Y 0 =) (Y 0)? ½ Y ?; Z ½ Z0 =) :?(Z0) ½ :?Z; (c) (?(Y ?))? = Y ?, :?((?Z)?) =? Z; (d) f0g? = X¤, X? = f0g, :?f0g = X. Proof. This is straightforward. Theorem 1.2.3. Let M be a subspace of X. Then (a) X¤=M ? =» M ¤; (b) If M is closed then (X=M)¤ =» M ?; (c) :?(M ?) = cl M. Proof. See [Go, p.25]. Theorem 1.2.4. If T 2 B(X; Y ) then (a) T (X)? = (T ¤)¡1(0); (b) cl T (X) = :?(T ¤¡1(0)); (c) T ¡1(0) ½ :?T ¤(Y ¤); (d) cl T ¤(Y ¤) ½ T ¡1(0)?. Proof. See [Go, p.59]. 8 CHAPTER 1. FREDHOLM THEORY Theorem 1.2.5. Let X and Y be Banach spaces and T 2 B(X; Y ). Then the fol- lowings are equivalent: (a) T has closed range; (b) T ¤ has closed range; (c) T ¤(Y ¤) = T ¡1(0)?; (d) T (X) = :?(T ¤¡1(0)). Proof. (a) , (d): From Theorem 1.2.4 (b). (a) ) (c): Observe that the operator T ^ : X=T ¡1(0) ! TX defined by x + T ¡1(0) 7! T x is invertible by the Open Mapping Theorem. Thus we have ¡ ¢¤ T ¡1(0)? =» X=T ¡1(0) =» (TX)¤ =» T ¤(Y ¤): (c) ) (b): This is clear because T ¡1(0)? is closed. ¤ ¤ ¤ (b) ) (a): Observe that if T1 : X ! cl (TX) then T1 : (cl TX) ! X is one-one. ¤ ¤ ¤ ¤ ¤ Since T (Y ) = ran T1 , T1 has closed range. Therefore T1 is bounded below, so that T1 is open; therefore TX is closed. Definition 1.2.6. If T 2 B(X; Y ), write ®(T ) := dim T ¡1(0) and ¯(T ) = dim Y=cl (TX): Theorem 1.2.7. If T 2 B(X; Y ) has a closed range then ®(T ¤) = ¯(T ) and ®(T ) = ¯(T ¤): Proof. This follows form the following observation: T ¤¡1(0) = (TX)? =» (Y=T X)¤ =» Y=T X and T ¡1(0) =» (T ¡1(0))¤ =» X¤=T ¡1(0)? =» X¤=T ¤(Y ¤): 9 CHAPTER 1. FREDHOLM THEORY 1.3 Definitions and Examples In the sequel X and Y denote complex Banach spaces. Definition 1.3.1. An operator T 2 B(X; Y ) is called a Fredholm operator if TX is closed, ®(T ) < 1 and ¯(T ) < 1. In this case we define the index of T by the equality index (T ) := ®(T ) ¡ ¯(T ): In the below we shall see that the condition “ TX is closed ” is automatically fulfilled if ¯(T ) < 1. Example 1.3.2. If X and Y are both finite dimensional then any operator T 2 B(X; Y ) is Fredholm and index(T ) = dim X ¡ dim Y : indeed recall the “rank theorem” dim X = dim T ¡1(0) + dim T X; which implies index(T ) = dim T ¡1(0) ¡ dim Y=T X = dim X ¡ dim TX ¡ (dim Y ¡ dim TX) = dim X ¡ dim Y: Thus in particular, if T 2 B(X) with dim X < 1 then T is Fredholm of index zero. Example 1.3.3. If K 2 B(X) is a compact operator then T = I ¡ K is Fredholm of index 0. This follows from the Fredholm theory for compact operators. Example 1.3.4. If U is the unilateral shift operator on `2, then index U = ¡1 and index U ¤ = ¡1: With U and U ¤, we can build a Fredholm operator whose index is equal to an arbitrary prescribed integer. Indeed if · ¸ U p 0 T = : `2 © `2 ! `2 © `2; 0 U ¤q then T is Fredholm, ®(T ) = q, ¯(T ) = p, and hence index T = q ¡ p. 10 CHAPTER 1. FREDHOLM THEORY 1.4 Operators with Closed Ranges If T 2 B(X; Y ), write ¡ ¢ © ª dist x; T ¡1(0) = inf jjx ¡ yjj : T y = 0 for each x 2 X: (1.3) If T 2 B(X; Y ), we define ½ ¾ γ(T ) = inf c > 0 : jjT xjj ¸ c dist (x; T ¡1(0)) for each x 2 X : we call γ(T ) the reduced minimum modulus of T . Theorem 1.4.1. If T 2 B(X; Y ) then T (X) is closed () γ(T ) > 0: Proof.
Details
-
File Typepdf
-
Upload Time-
-
Content LanguagesEnglish
-
Upload UserAnonymous/Not logged-in
-
File Pages207 Page
-
File Size-