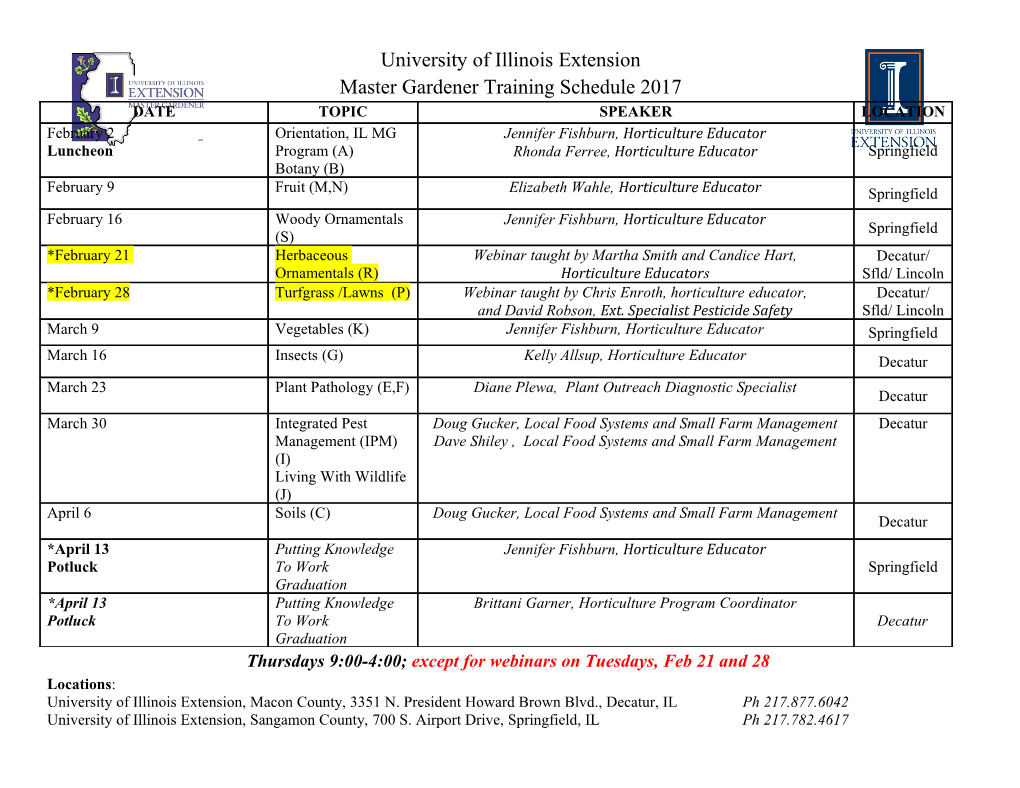
191 NMR and plant metabolism Richard Bligny and Roland Douce* Recent advances in NMR methodology offer a way to acquire (Figure 1). NMR experiments using 13C-labelled com- a comprehensive profile of a wide range of metabolites from pounds to decipher a metabolic pathway are usually various plant tissues or cells. NMR is a powerful approach for hampered by the low sensitivity of the 13C nucleus (which plant metabolite profiling and provides a capacity for the is four-fold lower than that of the 31P nucleus). This dynamic exploration of plant metabolism that is virtually problem can be overcome by using the cyclic j-cross unmatched by any other analytical technique. polarisation technique, which allows the indirect detection of 13C nuclei coupled to 1H nuclei by exploiting the high Current Opinion in Plant Biology 2001, 4:191–196 NMR sensitivity of protons [5]. The signal provided by the sensitive protons theoretically increases the signal/noise 1369-5266/01/$ — see front matter ratio by a factor of 64 over that of the 13C signal. It is also © 2001 Elsevier Science Ltd. All rights reserved. possible to enhance the 13C-signal using the nuclear Addresses Overhauser effect (i.e. a change in the intensity of a spec- Département de Biologie Moléculaire et Structurale, Laboratoire de troscopic signal caused by the irradiation of another Physiologie Cellulaire Végétale, CEA, CNRS et Université Joseph [ •] Fourier, 17 rue des martyrs, F 38054 Grenoble, Cedex 9, France nucleus during the NMR experiment) 6 . *e-mail: [email protected] NMR signal intensities are important analytically because at Abbreviation NMR nuclear magnetic resonance a given pulse sequence (signals are elicited by pulses of radiofrequency irradiation) they can be related to the con- tent within the tissue of the molecules that produce the Introduction signals. On the other hand, the chemical-shift effect ensures Nuclear magnetic resonance (NMR) spectroscopy can be that many metabolites, such as glucose, glucose-6-P and used to analyse the composition of tissues both in vivo and ATP, can be identified on the basis of their characteristic in various extracts. NMR has its origin in the net magnetic NMR signal patterns observed either in vivo (in a non-inva- moment or spin of an atomic nucleus that has an odd atom- sive way) or in vitro (in a perchloric extract). Moreover, the ic mass and/or an odd atomic number [1]. Common nuclei strength of NMR for tackling metabolism lies in the fact that that exhibit such magnetic properties are the highly abun- isotopic labels in different positions of different compounds dant isotopes 1H (99.98% in nature) and 31P (100% in can be simultaneously measured. nature) or the low abundance isotopes 13C (1.1% in nature) and 15N (0.37% in nature). The widespread use of NMR to In vivo NMR methods are frequently used to provide analyse plant metabolism and its compartmentation has information on the absolute concentrations of the more been reviewed recently [1,2,3••]. We have chosen to abundant metabolites, including sucrose, glucose 6-P and focus on restricted recent examples to convince the reader inorganic phosphate, and on how these concentrations that NMR is a powerful approach for plant metabolite change during biochemical transformation. These methods profiling [4••]. eliminate the need for extractions and sample preparation procedures. Several devices exist that are used to maintain NMR spectra a living system in a physiologically viable and controllable NMR signals (i.e. resonance) are observed when a sample state [1,2,3••]. These include the use of small leaf pieces is irradiated with pulses of radiofrequency electromagnetic that are infiltrated with perfusion medium, cell suspen- radiation in a strong magnetic field. Each nucleus within a sions at high cell density and root tips. These systems molecule experiences a slightly different magnetic field entail maintaining the supply of oxygen and various because of its distinct chemical environment and absorbs nutrients, including sugars, and the removal of waste energy at a slightly different frequency. The separation of products such as ethanol. By modifying the composition of these resonance frequencies from an arbitrarily chosen the circulating medium (e.g. by introducing phosphate or reference is called the chemical shift (δ), which is calculated amino acids, sucrose starvation or removing oxygen) it is on the basis of the relationship: possible to perturb the metabolism of the living system and to monitor the spectral changes caused by these δ = (resonance frequency of the sample [νs] – resonance changes simultaneously, thereby obtaining several succes- frequency of the reference [νr]) / (νr.106) sive spectra from the same sample. For example, aerobic and anaerobic conditions could be alternated at intervals of δ, which is independent of the field strength of the magnet, as short as 2 min. Examination of intact cells or tissues by is a unitless number that is expressed in parts per million NMR, however, gives relatively poor separation of signals (ppm). The result of an NMR analysis of a tissue is a spec- (i.e. allows more overlapping signals), loss of coupling multi- trum, that is, a plot of intensity (area) against chemical shift plicities (i.e. loss of J-coupling) and relatively poor in which each signal occurs at a characteristic energy signal-to-noise ratios. Hence, the detection and quantification 192 Physiology and metabolism Figure 1 vac-His 12 h (Incubation with 100 µ M histidine) C3 His C2 cyt-His His His C5 C3 12 h His His His C1 C4 C6 9 h O C 2 3 Sucrose HO 1 CHCH 2 4 5 6 h NH2 N N H Histidine 6 3 h cit mal cit 0 h (Reference spectrum) Glu Glu 0 h 180 160 140 120 100 80 60 40 20 34 32 30 28 26 Chemical shift (δ), ppm Current Opinion in Plant Biology 13 A pulse-chase experiment utilising in vivo C-NMR techniques. Series incubation medium contained 1 mM KCl, 5 mM KNO3, 0.5 mM of NMR spectra registered during the incorporation of histidine by MgSO4, 0.5 mM Ca(NO3)2, 0.1 mM KH2PO4, and 4 mM glucose. The heterotrophic culture sycamore cells. The spectra were recorded at pH of the external medium was regulated to 6.0. Histidine (100 µM) 20 °C on a Bruker AMX 400, WB, spectrometer, which was equipped was added at time 1 h; a chase was started at time 16 h (see Figure 2) with a 25-mm probe tuned at 161.9 MHz. They are the result of after the rinsing of perfused cells with a nutrient medium devoid of 900 scans (1 h). The signal-to-noise ratio was maximised using a histidine. The total height of the 12 sucrose peaks is not shown. specific perfusion arrangement [13•], which optimised the Citrate (cit), malate (mal) and glutamate (Glu) are also identified. The homogeneity of the analysed samples. Cells (10 g wet weight) were profile of soluble metabolites present in the reference spectrum of placed on a porous plate near the bottom of a 25-mm NMR tube. The sycamore cells shows that sucrose is the most abundant compound. porous plate was crossed by a central output glass tube; an inlet tube Quantification assays show that its concentration was circa 80 µmol/g and a safety output tube were positioned 2 cm above the surface of cell wet weight. The incorporated histidine appears as six pairs of the sedimented cells. The nutrient medium was circulated though the peaks corresponding to the six carbon atoms of the molecule. Except cells via a peristaltic pump attached to these tubes and recycled in a for carbon C5, the right peak in each pair corresponds to histidine well-oxygenated external reservoir. The conditions for 13C-NMR present in an acidic compartment (i.e. the vacuole at pH 5.7) and the acquisition utilised 70-µs pulses (90°) at 5.6-s intervals and a sweep left one to histidine present in an alkaline compartment (i.e. the width of 20.73 kHz. Broad-band decoupling at 4W during acquisition cytoplasm at pH 7.5). Carbon C5 splits in the opposite direction and 0.5 W during delay were applied using the Waltz sequence. according to pH shifts. cyt-His, cytoplasmic histidine; vac-His, Spectra were referenced to hexamethyldisiloxane at 2.7 ppm. The cells vacuolar histidine. of signals, especially of less intense signals, are typically from different elements is considerably reduced and inferior to those achieved using extracted tissue samples. coupling multiplicities are seen. In addition to one-dimen- For this reason, spectroscopy in vivo is usually supported sional NMR spectra from plant extracts, a wide range of by spectra taken from extracts of the cells or tissues being NMR-spectroscopic methods that generate two-dimen- investigated (i.e. from perchloric extracts that are devoid of sional spectra of different nuclei have now been paramagnetic cations such as manganese). Such extract developed. Indeed, a typical metabolite contains more spectra have sharp lines and hence overlapping of signals than one element that is detectable by NMR. NMR and plant metabolism Bligny and Douce 193 Furthermore, atoms of the same element within molecules Figure 2 are chemically non-equivalent and therefore worth distin- guishing from one another by NMR. The type of 20 additional information provided by 2D spectra and how it is interpreted is carefully described by Fan [7]. The various NMR spectroscopy techniques offer, there- Total (cyt+vac) fore, some unique ways to decipher metabolic networks in plants, to probe the metabolic response of tissues or cells to physiological [2,8–10,11•–13•] and chemical [14•] perturba- vac-His tions, to follow the fate of a stable-isotope-labelled molecule along a metabolic pathway [15–24,25•,26•], to visualise the unpredictable changes in metabolism reported mol/g cell wet wt) mol/g cell wet in genetically engineered plants [3••], and to analyse the µ 10 metabolic dialogue between plants and their microbial symbionts ([27]; see also Update).
Details
-
File Typepdf
-
Upload Time-
-
Content LanguagesEnglish
-
Upload UserAnonymous/Not logged-in
-
File Pages6 Page
-
File Size-