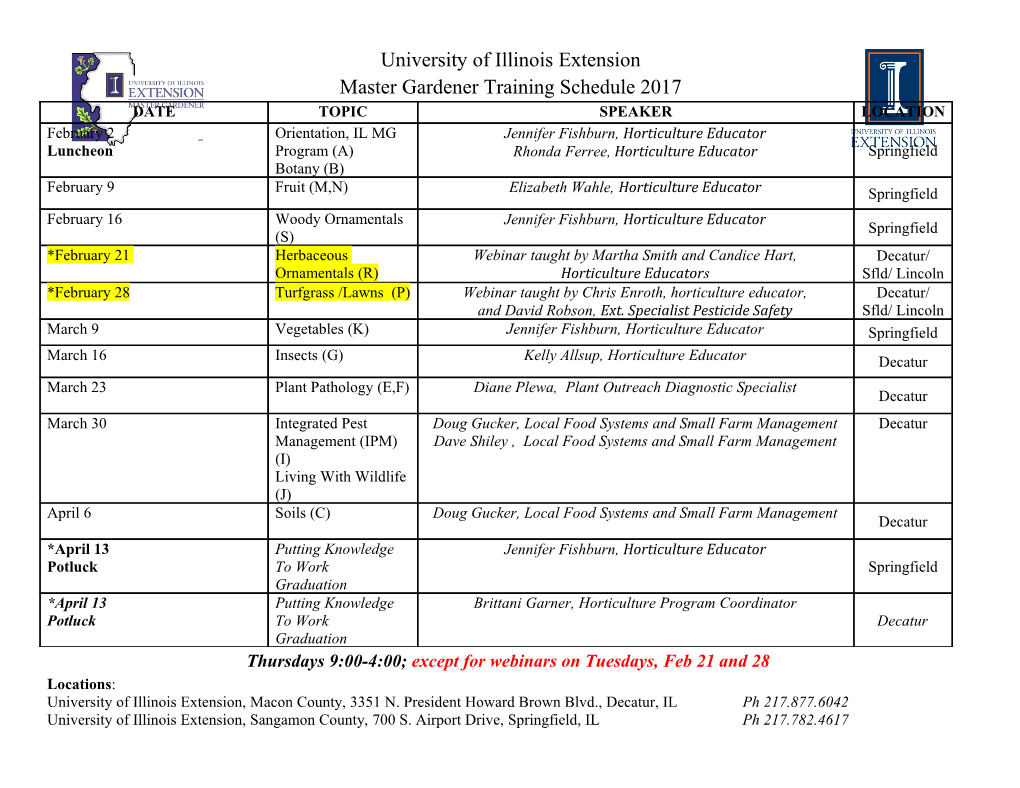
DUAL VECTOR SPACES AND SCALAR PRODUCTS JURGEN¨ TOLKSDORF NOVEMBER 28, 2012 1. Dual vector spaces Let, respectively, V and W be a real vector spaces of dimension 1 ≤ n < 1 and 1 ≤ m < 1. The real vector space of all linear maps from V to W is denoted by m×n HomR(V; W ). It is isomorphic to R when a basis is chosen in V and W . Definition 1.1. The real vector space ∗ V := HomR(V; R) ≡ fα : V −! R j linear g (1) of all linear maps from V to R (the latter considered as a real one-dimensional vector space) is called the dual vector space of V . Lemma 1.1. The dimension of V ∗ equals the dimension of V . ∗ Proof. Let ~v1; : : : ;~vn 2 V be a basis. One may define n linear maps νi 2 V as νi(~vj) := δij (1 ≤ i; j ≤ n) : (2) Remember that any linear map is fully determined by its action on an (arbitrary) P basis. In fact, for ~v = 1≤k≤n λk~vk one gets νi(~v) = λi 2 R (i = 1; : : : ; n). ∗ We prove that ν1; : : : ; νn 2 V are linearly independent. Assume that the vector P ∗ α := 1≤k≤n µkνk 2 V is the zero map. I.e. ν(~v) = 0 2 R holds for all ~v 2 V . Since this holds for all ~v 2 V , it follows that µ1 = µ2 = ··· µn = 0 simply by letting ∗ ~v = ~vk; k = 1; : : : ; n. Hence, n ≤ dim(V ). ∗ To demonstrate that span(ν1; : : : ; νn) = V , we remember that the vector space HomR(V; W ) of all linear maps between real vector spaces V and W (each of finite dimension) equals dim(V )dim(W ). In fact, choosing a basis in V and a basis in W dim(V ) dim(W ) allows to identify V with R and W with R . Hence, HomR(V; W ) can be identified with the real vector space Rdim(V )×dim(W ) of dimension dim(V )dim(W ). In ∗ the special case where W = R one therefore obtains that dim(V ) = dim(V ) as was to be proven. Notation: The elements of V ∗ are referred to as linear functionals, or one-forms. They are also called \dual vectors" or, especially in physics, as \co-vectors". Accord- ∗ ingly, the basis ν1; : : : ; νn 2 V that is defined by (2) is called the dual basis (co-basis) of the basis ~v1; : : : ;~vn 2 V . Notice that the dual basis of some chosen basis ~v1; : : : ;~vn in V is uniquely defined by ~v1; : : : ;~vn 2 V . Furthermore, in general it only makes sense to speak about the dual of a basis. In contrast, with a single vector ~v 2 V one cannot, in general, associate a (uniquely determined) dual vector α 2 V ∗. 1 2 J. TOLKSDORF n n Example: We may consider V = R with its standard basis ~e1; : : : ;~en 2 R . Its dual basis is simply defined by the mappings: ∗ n ek : R −! R (3) (x1; x2; : : : ; xn) 7! xk (k = 1; : : : ; n) : n When the real vector space R of n−tuples is identified with the real vector space n×1 n ∗ R of matrices of size n × 1, the dual space (R ) is identified with the real vector 1×n space R of matrices of size 1 × n. That is, one has the isomorphism t n ∗ 1×n σn :(R ) −! R X ∗ (4) α = µkek 7! µ ≡ (µ1; : : : ; µk) ; 1≤k≤n P n such that for all ~y ≡ (y1; : : : ; yn) = 1≤k≤n yk~ek 2 R and 0 1 y1 B . C n×1 y ≡ σn(~y) := @ . A 2 R (5) yn n ∗ n one obtains for the action of α 2 (R ) on R that X α(~y) = µy = µkyk 2 R : (6) 1≤k≤n ∗ ∗ In particular, ei (ej) = ei ej = δij for all 1 ≤ i; j ≤ n. 1×n n As a warning to not confound R with R we discuss the case n = 2 in some ~ 2 ~ 2 detail. For this consider b1 := ~e1 + ~e2 = (1; 1) 2 R and b1 := ~e1 − ~e2 = (1; −1) 2 R 2 ~ ~ as a different basis in R . It follows that ~x ≡ (x1; x2) = x1~e1 + x2~e2 = y1b1 + y2b2 = 2 2×1 (y1 + y2; y1 − y2) 2 R . The coordinate vectors x; y 2 R read: x y x = 1 ; y = 1 : (7) x2 y2 2×1 n Here, the coordinate vector x 2 R represents ~x 2 R with respect to the standard n 2×1 basis ~e1;~e2 2 R . In contrast, the coordinate vector y 2 R represents the same 2 ~ ~ 2 ~x 2 R with respect to the non-standard basis b1; b2 2 R . ∗ ∗ 2 ∗ ~ ~ 2 How does the dual basis b1; b2 2 (R ) of the basis b1; b2 2 R look like? For this one has to solve the linear system of equations that corresponds to the definition of a ∗ ~ dual basis bi (bj) := δij ; 1 ≤ i; j ≤ 2: ∗ ∗ b1(1; 1) = 1 ; b1(1; −1) = 0 ; ∗ ∗ (8) b2(1; 1) = 0 ; b2(1; −1) = 1 : ∗ P ∗ 2 ∗ Clearly, we may write bi = 1≤j≤n βijej 2 (R ) for i = 1; 2. Notice the order of the indices and compare this with the corresponding expansion 2 of the non-standard basis with respect to the standard basis of R . EUCLIDEAN SPACES 3 One obtains the system of linear equations β11 + β12 = 1 ; β11 − β12 = 0 ; (9) β21 + β22 = 0 ; β21 − β22 = 1 ; whose unique solution is given by β11 = β21 = β21 = −β22 = 1=2. Hence, ∗ 1 ∗ ∗ b1 = e1 + e2 ; 2 (10) 1 b∗ = e∗ − e∗ : 2 2 1 2 ∗ ∗ ∗ ∗ 2 ∗ The corresponding matrix representations of α = µ1b1 + µ2b2 = λ1e1 + λ2e2 2 (R ) 1×2 1×2 read: µ = (µ1; µ2) 2 R and λ = (λ1; λ2) 2 R . That is to say, both dual basis are represented by the same matrices: ∗ t ∗ t ∗ ∗ 1×2 e1 ≡ σ2 (e1) = σ2 (b1) ≡ b1 = (1; 0) 2 R ; (11) ∗ t ∗ t ∗ ∗ 1×2 e2 ≡ σ2 (e2) = σ2 (b2) ≡ b2 = (0; 1) 2 R : It follows that α(~x) = λx = µy 2 R : (12) Indeed, one has µy = µ1y1 + µ2y2 1 1 = (λ + λ )(x + x ) + (λ − λ )(x − x ) 2 1 2 1 2 2 1 2 1 2 (13) = λ1x1 + λ2x2 = λx : n ∗ n ∗ Notice that ~ek 2 R , as opposed to ek 2 (R ) . It is the matrix representation ∗ 1×n ∗ n ∗ n ek 2 R of ek 2 (R ) that looks formally identical to ~ek 2 R . This is because real n−tuples and real matrices of size 1×n look alike. As the example demonstrates, how- n ∗ ever, the matrix representation of any basis in (R ) formally looks like the standard n n basis of R . Therefore, one has to clearly distinguish between ~x = (x1; : : : ; xn) 2 R ∗ 1×n ∗ and x = (x1; : : : ; xn) 2 R . In the latter case, x is supposed to represent some n ∗ n element in (R ) with respect to some chosen basis. In contrast, ~x = (x1; : : : ; xn) 2 R does not refer to any such a choice of basis. n ∗ Furthermore, a linear form (co-vector) α 2 (R ) can never be expressed in terms n of an n−tuple, though its action on R can be represented by matrix multiplication: 1×n n ∗ α(~v) = αv. The matrix α 2 R represents the linear form α 2 (R ) and the matrix n×1 n ~ ~ n v 2 R the vector ~v 2 R with respect to a chosen basis b1;:::; bn 2 R and its ∗ ∗ n ∗ corresponding dual basis b1; : : : ; bn 2 (R ) . The value λx 2 R is independent of the choice of the basis used to express α(~x) in terms of matrix multiplication. This is most clearly expressed, for instance, by the equality (12). To see why this this holds true, actually, let ~v1; : : : ;~vn 2 V be any ∗ ∗ ∗ basis with its dual basis denoted by v1; : : : ; vn 2 V . Also, let ~u1; : : : ; ~un 2 V be ∗ ∗ ∗ another basis with corresponding dual basis u1; : : : ; un 2 V . Let f 2 AutR(V ) be an isomorphism that is defined by f(~vk) := ~uk for all k = 1; : : : ; n ≡ dim(V ) ≥ 1. 4 J. TOLKSDORF ∗ ∗ ∗ Correspondingly, let g 2 AutR(V ) be an isomorphism on the real vector space V ∗ ∗ ∗ ∗ that is defined by g (vk) := uk for all k = 1; : : : ; n = dim(V ). We claim that g∗ is fully determined by f −1. Indeed, from the definition of a dual basis one infers that ∗ ∗ ∗ ∗ ui (~uj) = g (vi ) f(~vj) = δij = vi (~vj) ; for all i; j = 1; : : : ; n : (14) P ∗ P ∗ We may rewrite this as follows. Let ~uj = 1≤i≤n aij~vi 2 V and ui = 1≤j≤n bijvj 2 ∗ V , with uniquely determined aij; bij 2 R for all 1 ≤ i; j ≤ n. It follows that for all 1 ≤ i; j ≤ n: ∗ X ∗ ui (~uj) = bikaljvk(~vl) 1≤k;l≤n X = bikaljδkl 1≤k;l≤n (15) X = bikakj 1≤k≤n = δij : n×n ∗ Therefore, the matrix B := (bij)1≤i;j≤n 2 R , representing the linear map g , must n×n be the inverse of the matrix A := (aij)1≤i;j≤n 2 R that represents the linear map f, i.e. B = A−1. Hence, the isomorphism g∗ is fully determined by f −1. To also demonstrate this more explicitly, we mention that every linear map g : V −! W between real vector spaces V and W uniquely determines a correspondingly linear map g∗ : W ∗ −! V ∗, via g∗(α)(~v) := α(g(~v)), for all α 2 W and ~v 2 V .
Details
-
File Typepdf
-
Upload Time-
-
Content LanguagesEnglish
-
Upload UserAnonymous/Not logged-in
-
File Pages9 Page
-
File Size-