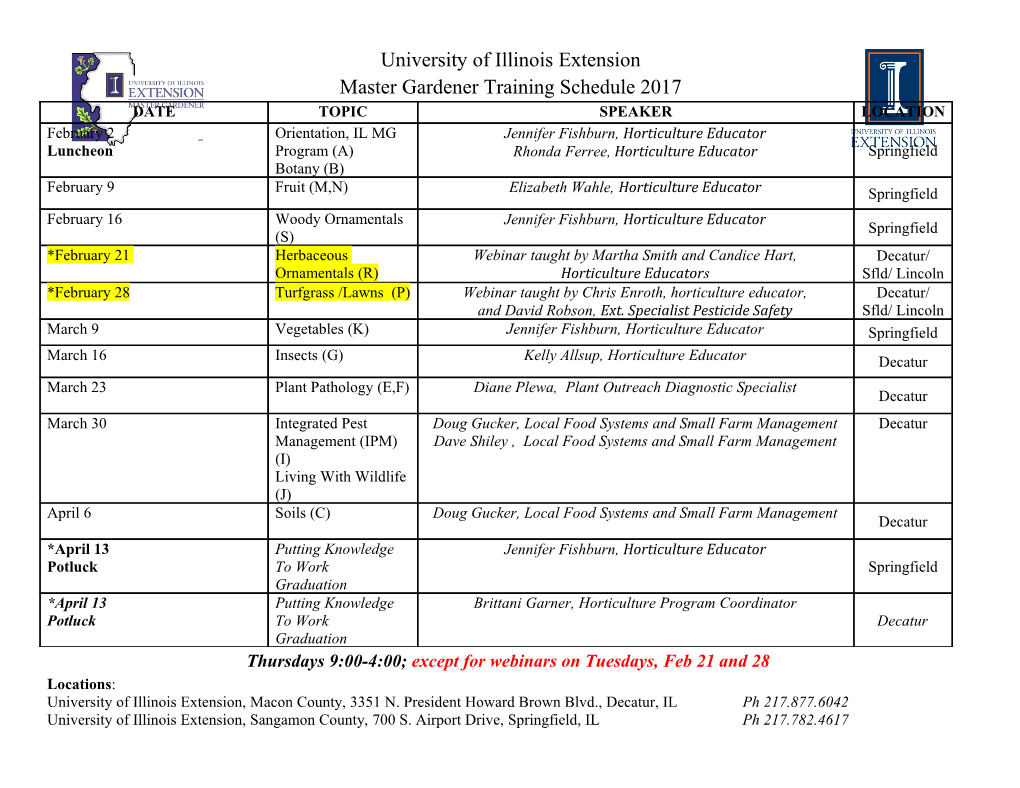
UC Irvine UC Irvine Electronic Theses and Dissertations Title Lithium 4,4’-Di-Tert-Butylbiphenylide: Characterization, Use in Spirocyclic Synthesis and Applications in Flow Reactors Permalink https://escholarship.org/uc/item/4zp5z2qm Author Hill, Richard Publication Date 2016 License https://creativecommons.org/licenses/by/4.0/ 4.0 Peer reviewed|Thesis/dissertation eScholarship.org Powered by the California Digital Library University of California UNIVERSITY OF CALIFORNIA, IRVINE Lithium 4,4’-Di-Tert-Butylbiphenylide: Characterization, Use in Spirocyclic Synthesis and Applications in Flow Reactors DISSERTATION Submitted in partial satisfaction of the requirements for the degree of DOCTOR OF PHILOSOPHY in Chemistry by Richard R. Hill Dissertation Committee: Professor Scott D. Rychnovsky, Chair Professor Kenneth J. Shea Professor Zhibin Guan 2016 © 2015 Richard R. Hill DEDICATION To my parents: Richard A. Hill and Melissa C. Hill ii TABLE OF CONTENTS Page LIST OF GRAPHS v LIST OF FIGURES vi LIST OF SCHEMES vii LIST OF TABLES x LIST OF ABBREVIATIONS xii ACKNOWLEDGMENTS xvi CURRICULUM VITAE xviii ABSTRACT OF THE DISSERTATION xx CHAPTER 1: Characteristics, Preparation and Stereoselectivity of α-Aminoorganolithiums I. Introduction 1 II. Reaction mechanisms of organolithium reagents 5 III. Generation of α-aminoorganolithiums 9 IV. Preparation by deprotonation 10 V. Preparation by lithium-halogen exchange 14 VI. Preparation by transmetallation 15 VII. Preparation by radical arene reduction 17 CHAPTER 2: LiDBB Formation and Decomposition Rates and Storage I. Introduction 28 II. Background 29 III. Titration studies 30 iii IV. Trapping studies 35 V. Rate studies 42 CHAPTER 3: Accessing Substituted Azaspirocycles Via Double Alkylation and Reductive Lithiation Methodology I. Introduction 57 II. Background 58 III. Project goals 62 IV. Synthesis of substituted dibromides 63 V. Optimization of double alkylation reaction 64 VI. Reductive lithiation to form [4.5] spirocycles 68 VII. Functional group tolerance of the LiDBB cyclization 74 VIII. Reductive lithiation to form [5.5] spirocycles 79 IX. Diagnostic nitrile 13C shifts 86 CHAPTER 4: Application of Flow Reactors to LiDBB Methodology I. Introduction 155 II. Background 155 III. Theory 162 IV. Epoxide ring opening in flow 166 V. Spirocycles in flow 174 iv LIST OF GRAPHS Page Graph 2.1 Rates of LiDBB formation 33 Graph 2.2 Relationship between [DBB] and [LiDBB] 34 Graph 2.3 Solution B from 0 °C to –25 °C 35 Graph 2.4 Solution A from 0 °C to –25 °C 35 Graph 2.5 Concentrations of Li+ from LiDBB decomposition 43 Graph 4.1 Expected rates of cooling to –78 °C at 30-second residence time 169 v LIST OF FIGURES Page Figure 1.1 Unstabilized and stabilized α-aminoorganolithiums 2 Figure 1.2 Conformational preference of α-aminoorganocarbanion and organolithium 2 Figure 1.3 Crystal and solution structures of α-aminoorganolithiums 3 Figure 1.4 Configurational stability of α-aminoorganolithiums and conducted tour 4 Figure 1.5 Electrophile approach for SE2ret or SE2inv mechanisms for 1.14 and 1.38 8 Figure 1.6 Common aromatic radical carriers 18, 30 Figure 3.1 Axial and equatorial Nitrile 13C shifts 86 Figure 4.1 Defining reactor design variables 163 Figure 4.2 Simple schematic of flow reactor with two syringe pumps 163 Figure 4.3 Conditioning of flow reactor tubing 170 Figure 4.4 Schematic of reactor 1 170 Figure 4.5 Schematic of reactor 2 176 Figure 4.6 Schematic of reactor 3 179 Figure 4.7 Schematic of reactor 4 182 vi LIST OF SCHEMES Page Scheme 1.1 Reaction mechanisms of organolithiums 6 Scheme 1.2 Stereochemical course of various electrophiles on 1.14 7 Scheme 1.3 Inversion and retention reactions of stabilized an α-aminoorganolithium 8 Scheme 1.4 Formation of alkyllithiums by permutational interconversion 9 Scheme 1.5 Formation of alkyllithiums by reductive insertion 10 Scheme 1.6 Alkylation of N–nitroso piperidines 12 Scheme 1.7 Trans-dialkylation of Boc protected piperidine 13 Scheme 1.8 Cis-dialkylation of N-formamidinyl piperidine 13 Scheme 1.9 Alkylation of a tertiary α-aminonitrile 14 Scheme 1.10 Stability and lithium-halogen exchange of α-aminoorganohalides 15 Scheme 1.11 Cyclization to (+)-pseudoheliotridane 15 Scheme 1.12 Anionic [2,3] rearrangement of pyrrolidium bromides 16 Scheme 1.13 Steric bias of oxazolidinone bearing α-aminoorganolithiums 17 Scheme 1.14 General mechanism of reductive lithiation 18 Scheme 1.15 Reductive lithiation of halobenzenes 19 Scheme 1.16 Decomposition pathways for LiN 19 Scheme 1.17 Decomposition pathway for LDMAN 20 Scheme 1.18 Early example of reductive decyanation on an α-aminonitrile 20 Scheme 1.19 Spiroannulation by reductive decyanation 21 Scheme 1.20 Reductive spiroannulation by carbolithation 22 Scheme 2.1 Reductive lithiation of an alkyl chloride 29 vii Scheme 2.2 Titration of LiDBB 31 Scheme 2.3 Known THF ring-opening reactions with organolithium anions and radicals 36 Scheme 2.4 LiDBB decomposition products identified by GCMS 37 Scheme 2.5 Synthesis of authentic samples of 2.9–2.11 37 Scheme 2.6 Synthesis of an authentic sample of DBB derivative 40 Scheme 2.7 Possible mechanism for forming compound 2.18 41 Scheme 2.8 Decomposition products of LiDBB in 2-methyltetrahydrofuran 42 Scheme 3.1 Azaspirocycles with pharmaceutical properties 58 Scheme 3.2 Alkylation and selective reductive lithiation of α-aminonitriles 59 Scheme 3.3 Key steps of (+)–lepadiformine C synthesis 60 Scheme 3.4 Protonation studies of lepadiformine C intermediate 61 Scheme 3.5 Reductive decyanation of 3.21 and 3.24 62 Scheme 3.6 General forward synthesis of spirocycles 63 Scheme 3.7 Synthesis of substituted dibromides 64 Scheme 3.8 Stereochemical outcome for phenyl containing spirocycle 69 Scheme 3.9 Stereochemical outcome for THP-containing spirocycle 70 Scheme 3.10 Reductive lithiation and protonation study on 3.39 71 Scheme 3.11 Possible explanation of stereochemical outcome of 3.61 72 Scheme 3.12 Synthesis of protected dibromides 75 Scheme 3.13 Possible decomposition mechanism for 3.95 78 Scheme 3.14 Attempts at selective deprotection of 3.100 79 viii Scheme 3.15 Simple [5.5] spirocycle synthesis 82 Scheme 3.16 Synthesis of methyl-substituted aminonitrile 3.123 and spirocycle 3.129 84 Scheme 3.17 Explanation of axial N-Boc in 3.39b 87 Scheme 4.1 Flow reactor set-ups for using dangerous reagents 159 Scheme 4.2 Self-optimizing flow reactor 160 Scheme 4.3 Preparation of key Amitriptyline intermediate via flow reactors 162 Scheme 4.4 Example flow reactor set-up 165 Scheme 4.5 Difference in yield of 4.25 based on pre-nucleophile source 167 Scheme 4.6 Expected elimination pathway for 4.23 168 Scheme 4.7 Preparation of authentic side products 3.120, 3.121, 4.36, 4.37 175 ix LIST OF TABLES Page Table 2.1 Sequential titrations of LiDBB 31 Table 2.2 Titrations with pretreatment 31 Table 2.3 Defining LiDBB solutions 32 Table 2.4 Trapping of vinyl lithium with m- tolualdehyde 39 Table 2.5 Conditions for the generation of ethylated DBB 40 Table 2.6 Reductive cyclizations with stored LiDBB 44 Table 3.1 Optimization of double alkylation of 3.11 with 3.29 65 Table 3.2 Synthesis of diastereomeric spirocycle precursors 67 Table 3.3 Synthesis of [4.5] spirocycles 73 Table 3.4 Synthesis of achiral/racemic [4.5] spirocycles 77 Table 3.5 Preparation of [5.5] spirocycles 81 Table 3.6 Temperature studies on product distribution from 3.119 83 Table 3.7 Methyl substituted [5.5] spirocycle temperature studies 85 Table 4.1 Equations and definitions for flow reactor theory 164 Table 4.2 Newton’s Law of cooling for flow reactor use 169 Table 4.3 Batch reactions of epoxide ring opening and additives 172 Table 4.4 Temperature vs. epoxide ring opening under flow conditions 173 Table 4.5 Varying residence time for opening epoxides 173 Table 4.6 Effect of residence time on yield of spirocycle 2.33 177 Table 4.7 Equivalents of LiDBB to form 2.33 in flow 178 Table 4.8 Non-cryogenic flow conditions to form a [5.5] spirocycle 180 x Table 4.9 Temperature dependence on diastereoselectivity of spirocycle 3.69 in flow 181 Table 4.10 Low molarity flow conditions to form a [5.5] spirocycle 183 Table 4.11 Low molarity flow conditions to form a [4.5] spirocycle 184 Table 4.12 Effect of residence time on diastereoselective spirocycle formation 185 Table 4.13 Mimicking flow reactions of 2.32 in batch 186 Table 4.14 Spirocycle 2.33 stability to LiDBB 187 xi LIST OF ABBREVIATIONS Å Angstrom(s) Ac Acyl Ar Aryl BPR Back pressure regulator Bn Benzyl Bz Benzoyl Bp Boiling point BF3•Et2O Boron trifluoride diethyl etherate Boc tert-butoxycarbonyl Bu Butyl CI Chemical ionization Cy Cyclohexyl DAST Diethylaminosulfur trifluroide dr Diastereomeric ratio DMF N,N-Dimethylformamide DMSO Dimethyl sulfoxide DMPU 1,3-dimethyl-3,4,5,6-tetrahydro-2(1H)-pyrimidinone DBB 4,4’-di-tert-butylbiphenyl EI Electron Impact ee Enantiomeric excess xii er Enantiomeric ratio Et Ethyl Equiv Equivalent FCC Flash column chromatography GCMS Gas chromatography-mass spectrometry GR Gas remover Hz Hertz HMPA Hexamethylphosphoramide HOMO Highest occupied molecular orbital HPLC High Performance Liquid Chromatography HRMS High resolution mass spectrometry IR Infared spectrometry i iso iPr isopropyl ID Internal diameter J Coupling constant KDBB Potassium 4,4’- di-tert-butylbiphenylide LDA Lithium diisopropylamide LDMAN Lithium 1-(dimethylamino)-naphthalenide LiDBB Lithium 4,4’- di-tert-butylbiphenylide LiN Lithium naphthalenide LiTMP Lithium tetramethylpiperidide MHz Megahertz xiii Me Methyl µ Micro m Milli Min Minute(s) M Molar NBS N-Bromosuccinimide NMI N-Methylimidazole
Details
-
File Typepdf
-
Upload Time-
-
Content LanguagesEnglish
-
Upload UserAnonymous/Not logged-in
-
File Pages229 Page
-
File Size-