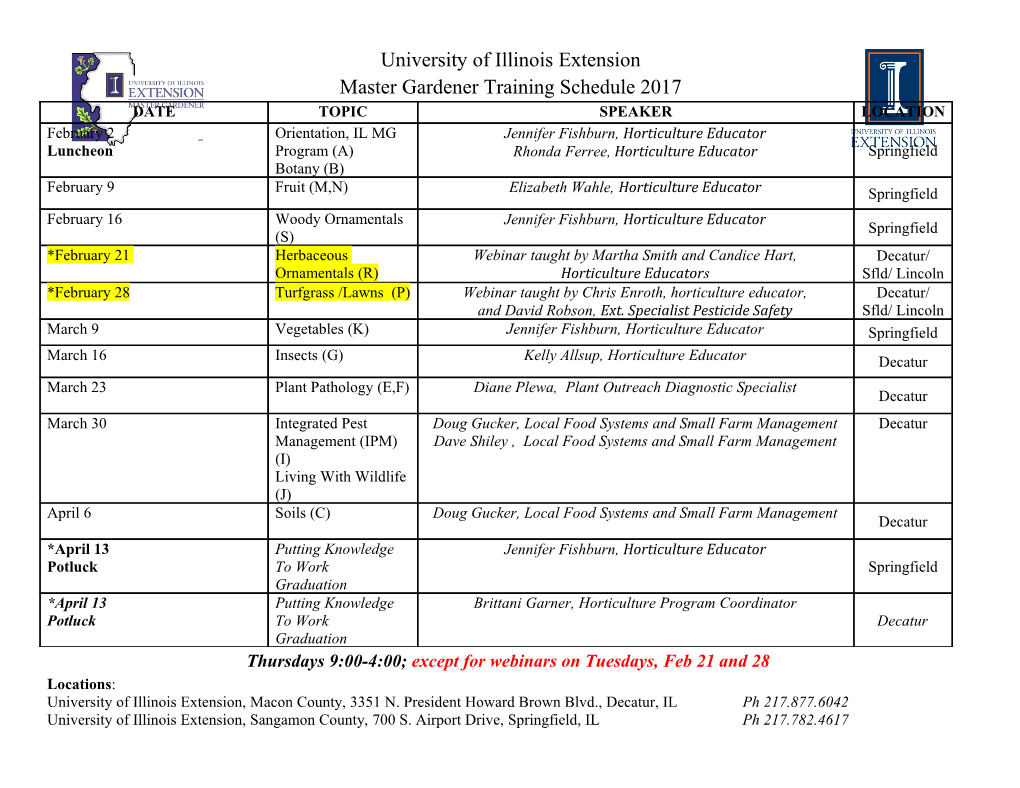
Localization of the Human Malaria Parasite’s Dihydrofolate Reductase-Thymidylate Synthase and Examining Its Role in Proguanil and Atovaquone Drug Synergy Joseph William Fowble A dissertation submitted in partial fulfillment of the requirements for the degree of Doctorate of Philosophy University of Washington 2014 Reading Committee: Pradipsinh K. Rathod, Chair Tomikazu Sasaki Robert E. Synovec Program Authorized to Offer Degree: Chemistry ©Copyright 2014 Joseph W. Fowble ii University of Washington Abstract Localization of the Human Malaria Parasite’s Dihydrofolate Reductase-Thymidylate Synthase and Examining Its Role in Proguanil and Atovaquone Drug Synergy Joseph W. Fowble Chair of the Supervisory Committee: Professor Pradipsinh K. Rathod Departments of Chemistry and Global Health Malaria is the common name for a disease caused by Plasmodium parasites. Malaria kills an estimated 1 million people out of approximately 200 to 500 million cases annually. The incredible number of cases of the disease, high numbers of parasites present in an infected individual, and sometimes rapid ability of the parasite to acquire drug resistance emphasizes the need to find new drugs to treat individuals and prevent infection. In the race to develop new drugs, elucidation of the exact mechanisms of older drugs and their targets is sometimes left unresolved. Dihydrofolate reductase (DHFR) has been an antimalarial drug target since the late 1940s, well before the rise of modern biochemistry and genetic analysis methods. Antimalarials targeting DHFR have been used for so long that resistant populations of parasites have rendered these antimalarials ineffective in many countries. While DHFR iii is among the oldest antimalarial drug targets identified, significant gaps remain in our understanding of the enzyme. Low levels of expression and essentiality in a haploid genome have made biochemical investigations of malarial DHFR and genetic manipulation particularly difficult. A few of the proteins proteins involved in pyrimidine biosynthesis and the folate cycle (dihydroorotate dehydrogenase (DHODH) and an isoform of serine hydroxymethyltransferase (SHMT)) have experimentally identified localizations that are mitochondrial. However, the localization of the malaria parasite’s conjoined dihydrofolate reductase and thymidylate synthase enzymes (DHFR-TS) has not been either investigated or reported. We pursued a theory that DHFR-TS is localized in the malaria parasite and that the observed in vitro synergy of Malarone®, combining an inhibitor of the mitochondrial electron transport chain and a pro-drug for a DHFR inhibitor, was influenced by DHFR inhibition and disruption of localization. The subcellular localization of the endogenous form of the DHFR-TS enzyme was investigated using the latest design techniques for peptide antigen-derived polyclonal antibodies, laser-scanning confocal microscopy, and advanced image analysis techniques interrogate the patterned DHFR-TS signal in relation to the organelles of the parasite. Comparisons between DHFR-TS and mitochondria-marking dyes, an engineered mitochondrial protein (HProtein-GFP), and native DHODH enzyme all indicate mitochondrial localization of this important drug target, DHFR-TS. Digitonin iv based selective permeabilization of cell membranes further illustrated that DHFR-TS is not cytosolic but localized and released alongside known mitochondrial protein. Proguanil and atovaquone drug synergy was evaluated with added consideration of DHFR localization involvement. Even with widespread resistance to antimalarials based on DHFR inhibitors, the modern antimalarial drug Malarone® combines atovaquone-based inhibition of the electron transport chain pathway with proguanil/cycloguanil-based inhibition of DHFR and the folate cycle. Atovaquone’s clinical trials were initially halted when it could not reliably cure patients due to the rapid acquisition of resistance to atovaquone. After proguanil was added to atovaquone a 98.7% cure rate was achieved, even in regions where DHFR-based drug resistance phenotypes were well established. We evaluated parasite strains with known DHFR mutations and observed Malarone® susceptibility in flasks largely matched the EC50 patterns predicted for DHFR- based inhibition by cycloguanil. We also identified parasite strains that displayed no synergistic interactions between proguanil and atovaquone. We analyzed parasite cultures treated with proguanil and atovaquone and, against the dogma of a human liver being necessary to create cycloguanil, observed low quantities of cycloguanil. In order to pursue a cycloguanil connection with minimal background genetic changes, a strain of parasites expressing a cycloguanil-resistant Plasmodium vivax DHFR-TS enzyme was created. The cycloguanil resistance conferred by the rescue plasmid did not translate into resistance to proguanil and atovaquone treatment with a transfection v construct that did not localize as the native DHFR-TS. We concluded that the rescue construct, without proper localization, was unable to fully replicate the function of the native falciparum DHFR-TS enzyme. vi Prior Publications Rathod Lab Narayanasamy K, Chery L, Basu A, Duraisingh MT, Escalante A, Fowble J, Guler JL, Herricks T, Kumar A, Majumder P, Maki J, Mascarenhas A, Rodrigues J, Roy B, Sen S, Shastri J, Smith J, Valecha N, White J, Rathod PK. (2012). "Malaria evolution in South Asia: knowledge for control and elimination." Acta Trop 121(3): 256-266. Guiguemde WA, Shelat AA, Bouck D, Duffy S, Crowther GJ, Davis PH, Smithson DC, Connelly M, Clark J, Zhu F, Jiménez-Díaz MB, Martinez MS, Wilson EB, Tripathi AK, Gut J, Sharlow ER, Bathurst I, El Mazouni F, Fowble JW, Forquer I, McGinley PL, Castro S, Angulo-Barturen I, Ferrer S, Rosenthal PJ, Derisi JL, Sullivan DJ, Lazo JS, Roos DS, Riscoe MK, Phillips MA, Rathod PK, Van Voorhis WC, Avery VM, Guy RK. (2010). "Chemical genetics of Plasmodium falciparum." Nature 465(7296): 311-315. Mui EJ, Schiehser GA, Milhous WK, Hsu H, Roberts CW, Kirisits M, Muench S, Rice D, Dubey JP, Fowble JW, Rathod PK, Queener SF, Liu SR, Jacobus DP, McLeod R. (2008). "Novel triazine JPC- 2067-B inhibits Toxoplasma gondii in vitro and in vivo." PLoS Negl Trop Dis 2(3): e190. Ganesan K, Ponmee N, Jiang L, Fowble JW, White J, Kamchonwongpaisan S, Yuthavong Y, Wilairat P, Rathod PK. (2008). "A genetically hard-wired metabolic transcriptome in Plasmodium falciparum fails to mount protective responses to lethal antifolates." PLoS Pathog 4(11): e1000214. Previous Labs Lockard JV, Zink JI, Luo Y, Weaver MN, Konradsson AE, Fowble JW, Nelsen SF. (2006). "Excited- state mixed-valence distortions in a diisopropyl diphenyl hydrazine cation." J Am Chem Soc 128(51): 16524-16531. Kulp SK, Yang YT, Hung CC, Chen KF, Lai JP, Tseng PH, Fowble JW, Ward PJ, Chen CS. (2004). "3- phosphoinositide-dependent protein kinase-1/Akt signaling represents a major cyclooxygenase- 2-independent target for celecoxib in prostate cancer cells." Cancer Res 64(4): 1444-1451. Zhu J, Huang JW, Tseng PH, Yang YT, Fowble J, Shiau CW, Shaw YJ, Kulp SK, Chen CS. (2004). "From the cyclooxygenase-2 inhibitor celecoxib to a novel class of 3-phosphoinositide- dependent protein kinase-1 inhibitors." Cancer Res 64(12): 4309-4318. vii Table of Contents CHAPTER 1 - BACKGROUND ..................................................................................................................... 1 MALARIA........................................................................................................................................................ 1 Global Burden of Malaria ....................................................................................................................... 1 History of Malaria ................................................................................................................................... 2 Species Differences/Distribution ............................................................................................................. 3 The Parasite Life Cycle ............................................................................................................................ 4 ESTABLISHED ANTIMALARIAL DRUG TARGETS ........................................................................................................ 6 Pyrimidine Biosynthesis and the Folate Cycle ......................................................................................... 7 Electron Transport Chain/Mitochondrial Function ............................................................................... 10 Heme Polymerization and Waste Disposal ........................................................................................... 11 Unclear Target – Artemisinin Family .................................................................................................... 12 THE MITOCHONDRION .................................................................................................................................... 13 Mitochondrial Localization Processes ................................................................................................... 14 Mitochondrial Localization Prediction Methods ................................................................................... 15 RESIDENT MITOCHONDRIAL FOLATE-UTILIZING ENZYMES ...................................................................................... 17 MALARONE® DEVELOPMENT ..........................................................................................................................
Details
-
File Typepdf
-
Upload Time-
-
Content LanguagesEnglish
-
Upload UserAnonymous/Not logged-in
-
File Pages170 Page
-
File Size-