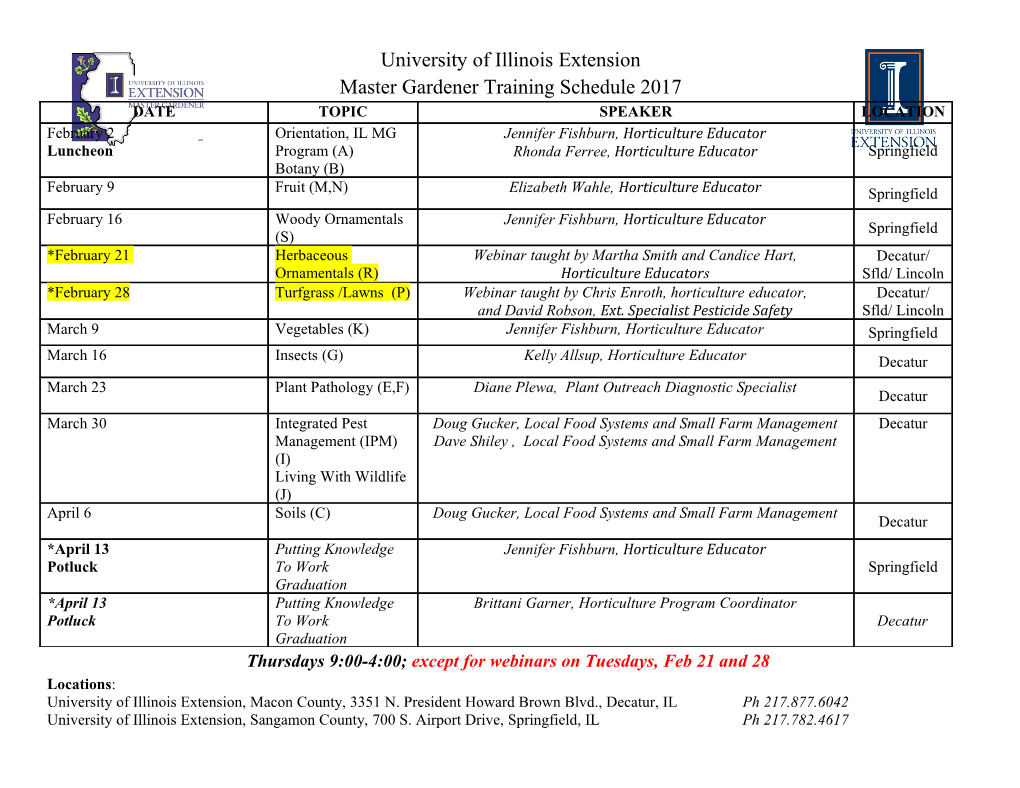
22 Recent Advances in Tropical Cyclogenesis Michael T. Montgomery Department of Meteorology, Naval Postgraduate School Monterey, California, USA [email protected] 1. Introduction The genesis of tropical cyclones, hurricanes and typhoons has been regarded by some as one of the most important unsolved problems in dynamical meteorology (Emanuel, 2005) and climate (Gore, 2006). From a scientific point of view, the problem is certainly a fascinating one. This article begins with a brief synopsis of the problem of tropical cyclogenesis and then presents an overview of some recent work on the tropical cyclogenesis problem by the author and his colleagues. The research reviewed here points to a unified view of the genesis and intensification process. It provides also a basis for some new tools to aid in forecasting tropical-cyclone formation. 1.1 Terminology Defined Before embarking on this review, it is appropriate to discuss some relevant terminology. The glossary on the Hurricane Research Division’s website uses “tropical cyclone as the generic term for a nonfrontal synoptic-scale low-pressure system over tropical or sub-tropical waters with organized convection (i.e. thunderstorm activity) and a definite cyclonic surface wind circulation (Holland, 1993).” Notably, this definition does not invoke any wind threshold. The same glossary defines a tropical depression as a tropical cyclone with maximum sustained surface winds of less than 17 m s-1 (34 kt, 39 mph) and, in the Atlantic and Eastern Pacific Basins, a “tropical storm” as a tropical cyclone with surface winds between 17 ms-1 and 33 ms-1. In contrast, a universally accepted definition of tropical cyclogenesis does not exist. For example, Ritchie and Holland (1999) define genesis as: “… the series of physical processes by which a warmcore, tropical-cyclone-scale 28 Michael T. Montgomery vortex with maximum amplitude near the surface forms”. In recent paper, Nolan et al. (2007) use a wind speed threshold of 20 ms-1 to define thetime of genesis. The formation of a tropical cyclone (TC), a phenomenon, commonly referred to as tropical cyclogenesis (hereafter, TC genesis), is a process by which some pre-existing, synoptic-scale or mesoscale weather feature in the tropics evolves so as to take on the characteristics of a tropical cyclone. The specific characteristics adopted by operational forecasting centres are as follows: a quasi-circular, closed circulation at or near the surface with the strongest circulating winds within or near the top of the atmospheric boundary layer and the presence of sustained deep moist convection near the centre of the circulation. For the purpose of this chapter, tropical cyclogenesis will be defined as the formation of a tropical depression as described above and, like Ritchie and Holland op. cit., but unlike Nolan, we impose no formal threshold on wind speed. We will refer to “intensification” as the amplification of the surface wind speed beyond the stage of tropical depression. Another issue, recognized long ago by Ooyama (1982), is the usage of the words “formation” and “genesis”. For the purposes of this chapter we will use these terms interchangeably. Moreover, it will be argued later that, from the point of view of understanding the formation and intensification process, a precise definition of cyclogenesis, understood as the attainment of specific wind thresholds, is unnecessary. 1.2 Necessary Conditions for Tropical Cyclogenesis Many disturbances travel across the tropical ocean basins during their respective tropical cyclone seasons, and yet relatively few become TCs. Since the pioneering work by Gray (1968), it has been known that a number of environmental conditions are favourable for genesis. For a cyclone to form six preconditions must be met: 1. Warm ocean waters (of at least 26.5°C) throughout a sufficient depth (unknown how deep, but at least on the order of 50 m). (Warm waters are necessary to fuel the heat engine of the tropical cyclone.) 2. An atmosphere which cools fast enough with height (is “unstable” enough) such that it encourages thunderstorm activity. (It is the thunderstorm activity that allows the heat stored in the ocean waters to be liberated for the tropical cyclone development.) 3. Relatively moist layers near the mid-troposphere (5 km). (Dry mid levels are not conducive for allowing the continuing development of widespread thunderstorm activity.) 4. A minimum distance of around 500 km from the equator. (Some of the earth’s spin is generally needed to provide a background rotation that can subsequently be concentrated and amplified through the partial material conservation of absolute angular momentum. Systems can form closer to the equator, or even on the equator given a disturbance with sufficient local vertical rotation, but these are relatively rare events.) Recent Advances in Tropical Cyclogenesis 29 5. A pre-existing disturbance near the surface with sufficient spin (vorticity) and inflow (convergence). (Tropical cyclones are generally not observed to form spontaneously. To develop, they require a weakly organized system with sufficient spin and low level inflow.) 6. Little change in the horizontal wind with height (low vertical wind shear, i.e. typically less than 40 km/h from surface to tropopause). (Large values of wind shear tend to disrupt the organization of the thunderstorms that are important to the inner part of a cyclone.) Having these conditions met is necessary albeit insufficient since many disturbances that appear to have favourable conditions do not develop. 1.3 The Forecasting Problem Over the past few decades there have been significant strides in improving tropical cyclone forecasting. Two formidable challenges that remain for TC forecasters are predicting TC intensity changes and forecasting TC genesis. The problem of forecasting TC genesis remains difficult for a number of reasons: (1) The structure and three-dimensional flow fields associated with pre-genesis systems are poorly understood, in part due to the relative lack of reconnaissance aircraft data and research campaigns compared to mature TCs; (2) TC genesis is often not well handled by operational forecast numerical models; and (3) there are competing theories for the fundamental processes involved, that culminate in the transformation of a cluster of thunderstorms into an organized tropical cyclone (Tory and Frank, 2010). 1.4 “Top down” Versus “Bottom up” Views There are two main viewpoints that have arisen to the forefront of the TC genesis discussion in the last two decades. The first includes two distinct variations, and they will be grouped together as “top-down”. Variation 1, or “Top-down merger” (Ritchie and Holland, 1997, 1993) features two mid-level vortices originating from the stratiform region of neighbouring mesoscale convective systems that interact, creating an area of enhanced cyclonic vorticity that appears to grow downwards. The downward growth of the merged circulation is a function of the local Rossby radius of deformation for asymmetric disturbances, NH/flocal, where N is the Brunt-Vaissalla frequency, H is an equivalent depth for internal gravity waves forced by deep convection and f = ()ff+ ζ ()+ 2vris the effective Coriolis parameter for the local tan local environment assuming weak departures of axisymmetric flow (Shapiro and Montgomery, 1993; Hoskins et al., 1985). According to this model, if the merged circulation extends to the surface it will promote the spin-up of an organized system that can result in the initiation of a tropical cyclone. Variation 2, or the “Top-down showerhead” theory of tropical cyclogenesis, was developed by Bister and Emanuel (1997) in their analysis of the data collected in the eastern Pacific basin during the TEXMEX experiment 30 Michael T. Montgomery conducted in the summer of 1991, based in Mexico. Unlike the model of Ritchie and Holland op. cit., this model requires only a single mesoscale convective vortex. This study emphasized the importance of thermodynamical processes within a so-called “mesoscale convective vortex embryo”. The study proposed that the development of a cool, moist environment resulting from stratiform rain serves as the incubation region for the formation of a low-level, warm- core cyclonic vortex. The study suggested that sustained precipitation in the stratiform cloud deck together with the evaporation of rain drops below would gradually cool and saturate the layer below cloud base while transporting cyclonic vorticity downwards to the surface. The idea is that there will be an accompanying increase in near-surface winds that would increase surface moisture fluxes leading ultimately to convective destabilization. A subsequent bout of deep convection was hypothesized to induce low-level convergence and vorticity stretching, thereby increasing the low-level tangential winds and “igniting” an amplification process fuelled by the increased surface moisture fluxes (the WISHE mechanism, see Chapter 21). Some questions about the dynamics of the pre-ignition process have been raised by Tory and Montgomery (2006) who noted, in particular, the inconsistency with vorticity substance impermeability between isobaric surfaces (Haynes and McIntrye, 1987). An equivalent way to understand this inconsistency is through the use of absolute angular momentum M (see Chapter 21). The downward advection of M by the downdraught is accompanied by horizontal divergence, which moves M surfaces outwards. The net effect of this process is one in which the M is materially conserved.
Details
-
File Typepdf
-
Upload Time-
-
Content LanguagesEnglish
-
Upload UserAnonymous/Not logged-in
-
File Pages28 Page
-
File Size-