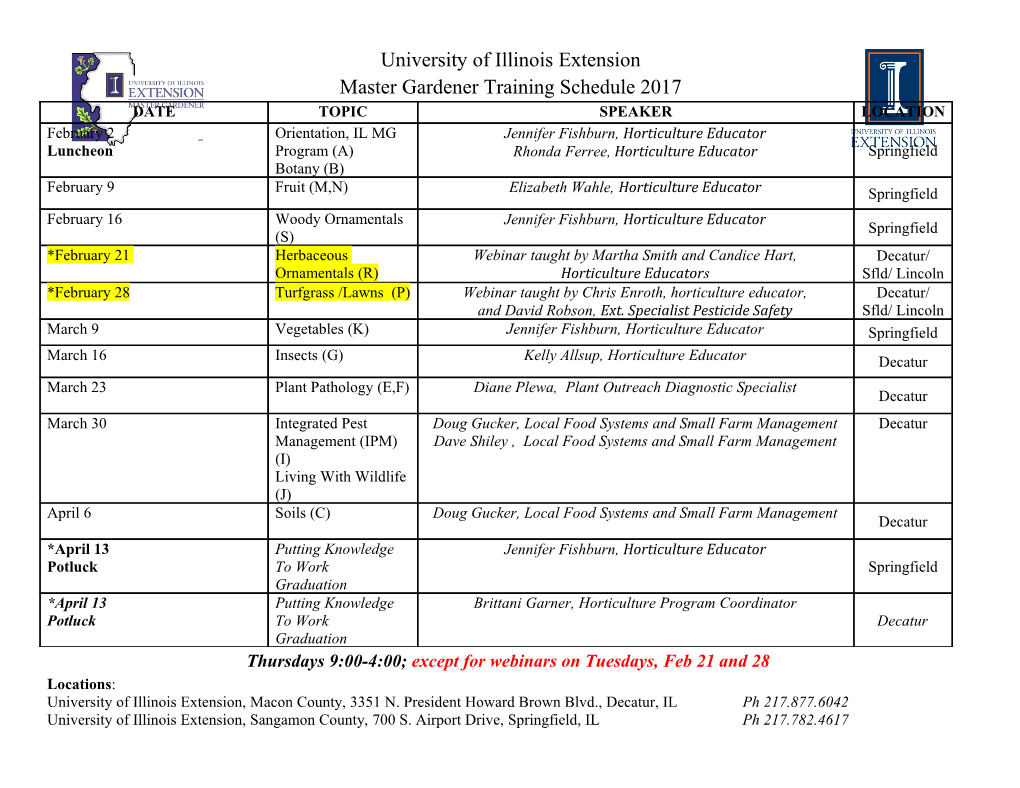
BIOIMAGING Research Report In situ imaging of mitochondrial outer- membrane pores using atomic force microscopy Bradley E. Layton, Ann Marie Sastry, Christian M. Lastoskie, Martin A. Philbert, Terry J. Miller, Kelli A. Sullivan, Eva L. Feldman, and Chia-Wei Wang BioTechniques 37:564-573 (October 2004) Here we describe a technique for imaging of the outer contours of the mitochondrial membrane using atomic force microscopy, subsequent to or during a toxic or metabolic challenge. Pore formation in both glucose-challenged and 1,3-dinitrobenzene (DNB)- challenged mitochondria was observed using this technique. Our approach enables quantification of individual mitochondrial membrane pore formations. With this work, we have produced some of the highest resolution images of the outer contours of the in situ mitochondrial membrane published to date. These are potentially the first images of the component protein clusters at the time of formation of the mitochondrial membrane transition pore in situ. With the current work, we have extended the application of atomic force microscopy of mitochondrial membranes to fluid imaging. We have also begun to correlate 3-D surface features of mitochondria dotted with open membrane pores with features previously viewed with electron microscopy (EM) of fixed sections. INTRODUCTION tion of a large, multiprotein porin com- channel size, and permeable substrates plex mediates either energy production for each membrane protein are shown Selective passage of solute mol- capability (closed probability state) or in Table 2 (14–22). It should be noted ecules into and out of the cell is ac- cell death (open probability state) by that while certain transport proteins complished by several classes of pore- preventing or permitting the exchange such as gramicidin form cylindrical forming proteins that are integral to the of matrix components with matrix and/ channels that are well described by a cell membrane. An important subset of or intermembrane components. Such single measure of internal pore width, these channel-forming proteins are the exchange is critical to secondary cel- many channel-forming proteins have porins (1), proteins that adopt a charac- lular processes (6–8) and thus largely internal pore cross sections that vary teristic barrel-shaped configuration and determines cell fate (9–13). Dozens of along the channel axis, with one or form channels spanning the extracellu- membrane channels have been identi- more internal cavities separated from lar and intracellular environments (2,3) fied to date, as summarized in Table 1 extracellular and intracelluar vestibules as shown in Figure 1. Porins are found (Reference 1; a database of these chan- by pore constrictions or gating regions. in the outer membranes of Gram-nega- nel-forming proteins may be found at In such instances, effective pore radii tive bacteria and in the membranes of http://saier-144-164.ucsd.edu/tcdb/). for the “cavity” and “gate” regions have mitochondria and chloroplast organ- Selected α-helix and β-barrel pore- been reported (Table 2) as obtained via elles in eukaryotic cells (1); typical forming proteins, with occurrence, crystallography (20) or from estimates interior diameters at the constriction obtained from permeation mea- regions within porin channels are about surements (21) or homology stud- 2 nm, which are sufficiently large to A B ies (22). allow the passage of a number of low One much studied porin is the molecular weight solutes via diffusive voltage-dependent, anion-selec- transport (4). Nonetheless, a large num- tive channel porin (VDAC). This ber of membrane porins are required porin is found in the mitochon- to accomplish significant transport. A drial outer membranes of animal typical bacterium, for example, is es- cells (23,24). It is selectively per- timated to have 105 porins in its outer meable to anions and can also un- membrane (5). Figure 1. OmpF, a trimeric β-barrel porin. (A) Top dergo a transition to be selective view, and (B) side view. In panel B, the top of the protein Porins play a critical role in cellular opens to the extracellular domain, and the bottom of the to cation transport (9). The VDAC and organellar transport processes. For protein opens to the intracellular domain. β strands are is also thought to play a key role example, in mitochondria, the forma- shown as ribbons and α-helices as red cylinders. in the regulation of mitochondrial- University of Michigan, Ann Arbor, MI, USA 564 BioTechniques Vol. 37, No. 4 (2004) Table 1. Classifications of Channel-Forming Membrane Proteins with Their Occurrence, The three-dimensional (3-D) shape Function, and a Representative Protein from Each Class of the VDAC pore has been determined Channel Type Cell/Organelle Type Function Example Protein to approximately 0.1 nm resolution (26), + using sequence analysis and circular di- α-helix bundle bacteria, animal, and communication, K channel chroism to find that the protein forms plant tissue cells, homeostasis mitochondria a structure similar to a bacterial porin- like β-barrel. Measured conductance of β-barrel porin gram-negative bacteria, general or specific OmpF porin a membrane consisting of VDAC pores mitochondria, transport across in a bilayer in the presence of molecules chloroplasts membrane with diameters on the order of the pore holin bacteria, viral induce apoptosis autolysin inner diameter (27) was used to deter- pathogens mine an inner diameter of 1.2–1.5 nm protein toxin bacteria, yeast, nutrient leakage colicin for the Neurospora crassa mitochon- protozoa drial VDAC pore. An overall molecular peptide toxin bacteria, animal cells disrupt membrane bee venom weight of 34 kDa has also been obtained via Western blot analysis (28,29). The mediated apoptosis. Specifically, it has preceding the known release of cyto- membrane has an approximate density been hypothesized to either coalesce chrome c and the resulting activation of of several thousand pores per square mi- into a megapore, the permeability tran- irreversible cascades of apoptotic, in- crometer, as measured in bovine heart sition pore (PTP), or alternatively, to tracellular “killer proteases” (e.g., Ref- with enzyme-linked immunosorbent as- close entirely, presumably resulting erence 25). Thus, both the opening and say (ELISA) (30), and in the neurospora in heightened internal mitochondrial closure of the pore and the dimensions fungus using conductance measure- pressure. Either hypothesis would be associated with each have been the sub- ments across membranes and using sin- consistent with mitochondrial rupture ject of intense study. gle-channel recordings (31). One of the BIOIMAGING Research Report Table 2. Selected α-Helix and β-Barrel Pore-Forming Proteins, with Occurrence, Channel Glucose Challenge Size, and Permeable Substrates for Each Membrane Protein Channel Organism Permeates Radius Reference Russell et al. (8) found that mitochon- (nm) dria from rat primary dorsal root ganglia + + + + (DRG) neurons challenged with 45 mM gramicidin bacteria H , Li , Na , K 0.2 Smart et al. (14) glucose for 6 h showed an increase in KcsA bacteria K+, Rb+, Cs+ 0.1 (gate) Doyle et al. (15) cross-sectional area of approximately 0.6 (cavity) Allen et al. (16) 50% (0.43 ± 0.03 μm2 vs. 0.66 ± 0.08 acetylcholine eukaryotes glycine, serotonin 0.3 (gate) Stein (17) μm2) by measuring maximal cross-sec- receptor 2.0 (cavity) Miyazawa et al. (18) tional area through a z-series of a fluo- OmpF bacterial outer nonselective for cat- 0.5 (gate) Jap et al. (19) rescent signals under confocal microsco- membrane ions, anions, sugars, 1.8 (cavity) Schirmer (20) py. We can estimate that this comprises others an 80% increase in volume, assuming an VDAC eukaryotic similar to OmpF, but 0.8 (gate) Benz (21) ellipsoidal shape for mitochondria (V ∝ mitochondrial voltage-dependent 1.3 (cavity) Casadio et al. (22) A3/2). Mitochondrial swelling was found anion selectivity to be dose- and time-dependent, with in- creased glucose molarities resulting in VDAC, voltage-dependent, anion-selective channel outer membrane porin. greater swelling rates, and longer expo- functional implications of its inherent cinoma cells. Several reports (43–45) sure resulting in greater volumetric in- pore geometry is that VDAC is readily have described 5 nm resolution images, creases (at 150 mM, 130% ΔA → 350% permeable to glucose, the latter of which obtained by imaging a relatively thick ΔV; at 300 mM, 166% ΔA → 440% has a diameter of <1 nm (32). (approximately 1 μm) section with ΔV) after 6 h. A plateau in cross-sec- Atomic force microscopy (AFM) a transmission electron microscope tional area (ΔA ~ 120% = ΔV ~ 225%) has been successfully applied to the (TEM) at a series of angles and recon- as measured by confocal microcopy was imaging of biological surfaces, as dem- structing the 3-D shape from the 2-D apparently reached after 24 and 48 h of onstrated in the characterization of the projections. excessive (45 mM) glucose exposure. general surface morphology of mito- In the present study, we use a re- Swelling appeared to be coupled with chondria (33–35), spermatozoa (36), fined AFM approach to investigate mi- the complete membrane depolarization and neurons (37). With its sub-nanome- tochondrial pore formation subsequent (loss of ΔΨmt) at 24 h, as measured by ter resolution and its capability to image to two specific types of challenge: ex- a change in wavelength of a voltage-de- features in aqueous environments, AFM cess glucose and 1,3-dinitrobenzene pendent fluorophore (8). is also well suited to imaging organelle (DNB) exposure. Under both condi- The precise mechanism for the dis- membrane proteins in situ (38) or in tions, mitochondria swell as a result of ruption of excess glucose on the viabil- monolayers (39,40). Examples of pre- water flux into the hypertonic matrix. ity of mitochondria is not yet known, but vious imaging experiments on the mi- Specific rationales follow for each likely involves disruption of the normal tochondrial outer-membrane structure case.
Details
-
File Typepdf
-
Upload Time-
-
Content LanguagesEnglish
-
Upload UserAnonymous/Not logged-in
-
File Pages9 Page
-
File Size-