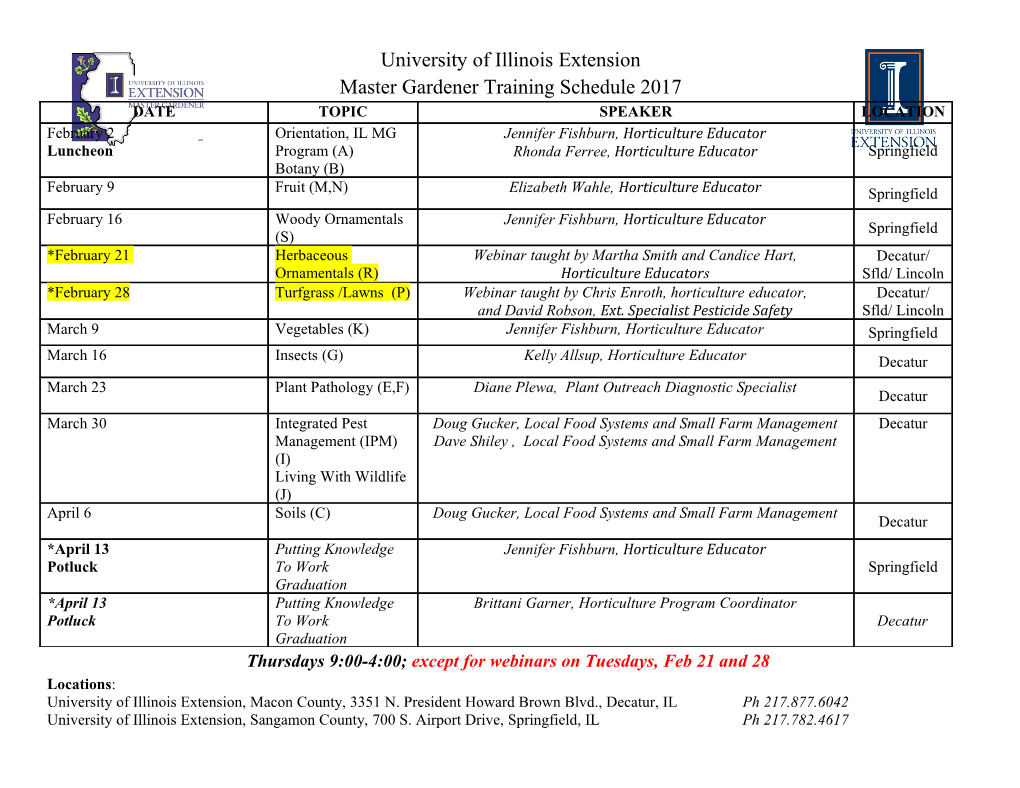
Edinburgh Research Explorer Leidenfrost droplets on microstructured surfaces Citation for published version: Duursma, G, Kennedy, R, Sefiane, K & Yong, Y 2016, 'Leidenfrost droplets on microstructured surfaces', Heat Transfer Engineering, vol. 37, no. 13-14, pp. 1190-1200. https://doi.org/10.1080/01457632.2015.1112610 Digital Object Identifier (DOI): 10.1080/01457632.2015.1112610 Link: Link to publication record in Edinburgh Research Explorer Document Version: Peer reviewed version Published In: Heat Transfer Engineering General rights Copyright for the publications made accessible via the Edinburgh Research Explorer is retained by the author(s) and / or other copyright owners and it is a condition of accessing these publications that users recognise and abide by the legal requirements associated with these rights. Take down policy The University of Edinburgh has made every reasonable effort to ensure that Edinburgh Research Explorer content complies with UK legislation. If you believe that the public display of this file breaches copyright please contact [email protected] providing details, and we will remove access to the work immediately and investigate your claim. Download date: 07. Oct. 2021 Leidenfrost droplets on microstructured surfaces Gail Duursma1*, Ross Kennedy1, Khellil Sefiane1,2, Yong Yu3 1School of Engineering, The University of Edinburgh, King’s Buildings, Mayfield Road, Edinburgh EH9 3JL, United Kingdom 2International Institute for Carbon-Neutral Energy Research(I2CNER), Kyushu University, 744 Motooka, Nishi-ku, Fukuoka 819-0395, Japan 3School of Aerospace Engineering, Beijing Institute of Technology, 5 South Zhongguancun Street, Haidian District, Beijing 100084, People's Republic of China *Address correspondence to Dr Gail Duursma, School of Engineering, The University of Edinburgh, The King’s Buildings, Mayfield Road, Edinburgh EH9 3JL, UK E-mail: [email protected] Phone Number: 0 (+44) 131 650 4868, Fax Number: 0 (+44) 131 650 6551 1 ABSTRACT The lifetime of a droplet deposited on a hot plate decreases when the temperature of the plate increases, but above the critical Leidenfrost temperature, the lifetime suddenly increases. This is due to the formation of a thin layer of vapour between the droplet and the substrate which plays a double role: first it thermally insulates the droplet from the plate and second it allows the droplet to “levitate.” The Leidenfrost point is affected by the roughness or microstructure of the surface. In this work, a silicon surface with different micro- structured regions of square-pillars was prepared such that there is a sharp transition (boundary) between areas of different pillar spacing. The Leidenfrost point was identified in experiments using water droplets ranging in size from 8 - 24 microlitres and the behaviour of the droplets was recorded using high-speed digital photography. The Leidenfrost point was found to vary by up to 120 °C for pillar spacings from 10 - 100 microns. If the droplet is placed on the boundary between structured sections, the droplet becomes asymmetric and may move or spin. An axisymmetric CFD model is also presented which shows qualitative agreement with experimental observations. Keywords: Leidenfrost, Boiling, Microstructure, Wettability . 2 INTRODUCTION The interaction of droplets with hot surfaces is relevant to a wide range of applications. When surfaces are above a critical temperature the droplets are observed to levitate. This latterly- called Leidenfrost effect was first reported in 1732 by Boerhaave [1] who noted that ethanol droplets deposited on superheated metal surfaces did not boil rapidly as expected and indeed persisted longer than droplets on cooler surfaces. In 1756, Leidenfrost made similar observations about the lifetime and mobility of water droplets deposited on a hot silver spoon in his paper, 'De Aquae' and gave his name to the phenomenon. The Leidenfrost effect can hamper cooling technologies in high heat flux applications and it is crucial for safety to avoid dryout in nuclear reactors, the consequences of which are catastrophic [2]-[3]. Other applications exploiting the Leidenfrost phenomenon are printing, jet impingement and vitrification of biofluids by levitation above liquid nitrogen – in this latter application the inhibition of heat transfer is beneficial as it produces the right freezing rate for cell preservation. Above the Leidenfrost Point (LFP), droplets begin to float on an insulating layer of their own vapour (see Fig. 1), resulting in a local maximum for droplet lifetime and a substantial increase in mobility, due to the large reduction in friction. The droplet levitation resulting from the Leidenfrost effect has been harnessed to direct droplet motion through mechanical macro-scale ratchets and has even been used to produce mazes [4]-[5]. In many industrial situations, operating in the Leidenfrost boiling regime is highly undesirable due to the inhibition to heat transfer. In metallurgical processes such as quenching and spray-cooling, the Leidenfrost effect inhibits the control of cooling by means of the insulating vapour layer. Fast cooling is necessary to maintain the mechanical strength of material, while uniform temperature profiles prevent deformation [6]-[7]. 3 The Leidenfrost effect has implications in both high heat flux microelectronics cooling systems and in fire-fighting emergencies where it is necessary for rapid heat removal. Indeed, accident reports following the Fukushima nuclear disaster have cited the inverse Leidenfrost effect as a contributor to the inability to cool the fuel system effectively while following emergency procedures [8]-[9]. Being able to alter the LFP of systems to either avoid or deliberately enter the Leidenfrost boiling regime is of particular interest across a wide variety of industrial applications. Being able to raise the LFP, by as much as 100 °C, can vastly extend the temperature range where Leidenfrost does not need to be considered for both process design and safety. Many properties such as thermal diffusivity and pressure are also shown to affect the Leidenfrost point, as shown in Orejon et al. [10]. Surface roughness has always been understood as a key parameter: measurements of the LFP on two surfaces of the same material have been shown to vary greatly depending on whether the material was polished or rough. [11]-[20]. Altering the Leidenfrost point may also be done by exploiting hydrophobicity of surfaces and/or structuring them. Liu and Craig [12] examined hydrophobic smooth flat substrates and found that Leidenfrost levitation occurred at lower substrate temperatures. Vakarelski et al. [11] used combinations of chemical modification and surface heterogeneity to stabilise vapour layers surrounding spherical substrates and avoid nucleate boiling. Recently, there has been particular interest in the influence of micro- and nano-structures being used to alter the LFP for enhanced boiling. Kwon et al. [16] carried out a systematic study of the effect of microstructures on the LFP of a water-silicon system over a range of micropillar parameters. The authors demonstrated experimentally that the Leidenfrost point could be shifted by as much as 100 °C by manipulating surface structure. They proposed a 4 mechanism to describe the variation of the LFP due to the presence of the microstructure array. They surmised that as the surface was highly wetting, there would be contact between the tops of the micropost array and the underside of the droplet, and that this contact would result in a force balance between the surface tension (Δpcap ~ ɣ/b) acting downwards and a vapour pressure (which can be modelled as radial Poiseuille flow) acting upwards. The authors suggest that the Leidenfrost state exists if the ratio of these competing vertical forces * balances is approximately unity; Δp vap / Δpcap ~ 1. On the other hand, modelling and simulation of these complex phenomena is sparse and most studies have been limited to flat surfaces. For instance, Rueda Villegas [21] has performed numerical simulations of the Leidenfrost effect using the level set method for droplets falling onto a smooth substrate. Using a numerical approach they were able to capture some aspects of this behaviour. Despite the numerous studies on the Leidenfrost phenomenon, recent findings on the significant effect of substrate roughness and structure demonstrate the need for further investigation of the phenomena. In the present study an experimental investigation of the effect of size and spacing of microstructures on Leidenfrost point is undertaken. Furthermore a numerical model is presented to describe the experimental observations. EXPERIMENTAL APPARATUS and PROCEDURES A silicon micro-structured surface was produced using deep etching. A 4 inch silicon wafer was treated with a photoresist polymer before standard lithography and etching techniques were used to impose the design, through a glass mask, onto the surface. The surface was post- processed with plasma oxidation to remove any residue from the etching process and to ensure that droplets deposited onto its surface are completely wetting (superhydrophilic). The microstructure design pattern imprinted onto the wafer is illustrated in Figure 2. All pillars 5 are square-topped; arranged in a grid-like array and have a uniform height of 10 µm, a height sufficient, it is felt, not to be a significant influence on wetting. The wafer (as shown in Figure 2) was divided into sections, each of which was created with a unique pillar thickness
Details
-
File Typepdf
-
Upload Time-
-
Content LanguagesEnglish
-
Upload UserAnonymous/Not logged-in
-
File Pages39 Page
-
File Size-