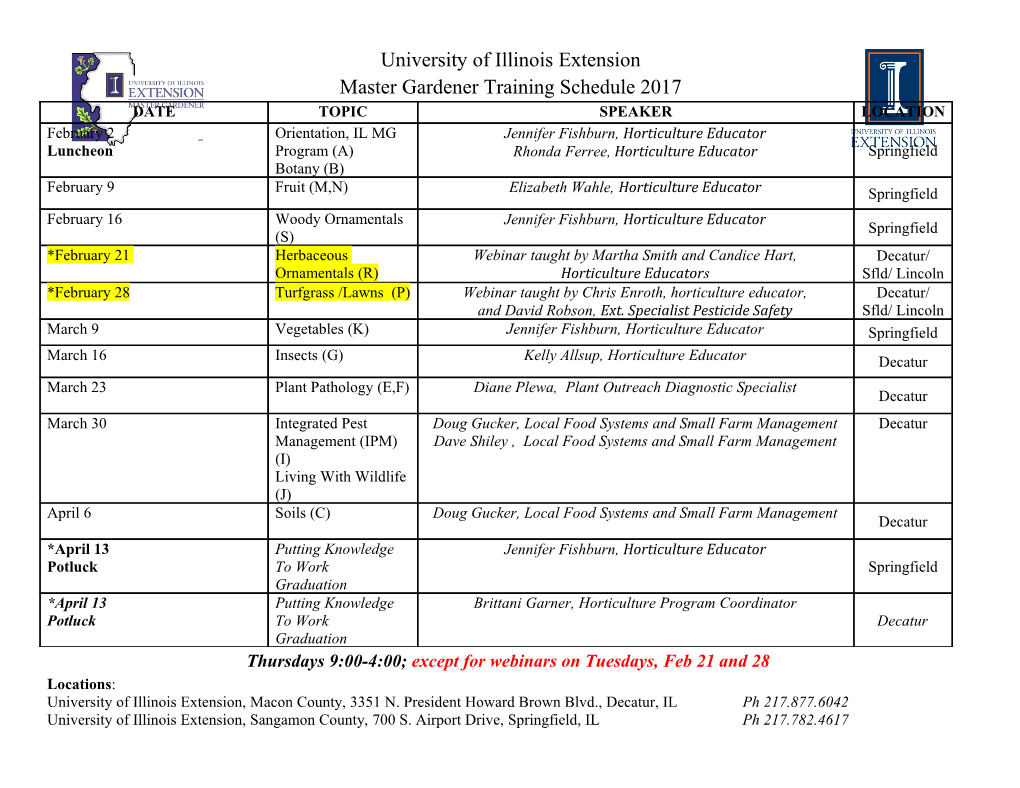
Nanophotonics 2021; 10(6): 1621–1655 Review Alexander Al-Zubeidi, Lauren A. McCarthy, Ali Rafiei-Miandashti, Thomas S. Heiderscheit and Stephan Link* Single-particle scattering spectroscopy: fundamentals and applications https://doi.org/10.1515/nanoph-2020-0639 imaging techniques used to extract spectral information. Received December 4, 2020; accepted February 16, 2021; Finally, we provide a brief overview of specialized setups published online March 8, 2021 for in situ measurements of nanoparticles in liquid systems and setups coupled to scanning tip microscopes. Abstract: Metallic nanoparticles supporting a localized surface plasmon resonance have emerged as promising Keywords: dark-field; hyperspectral; nanoparticle; platforms for nanoscopic labels, sensors, and (photo-) plasmon; polarization. catalysts. To use nanoparticles in these capacities, and to gain mechanistic insight into the reactivity of inherently heterogeneous nanoparticles, single-particle character- 1 Introduction ization approaches are needed. Single-particle scattering spectroscopy has become an important, highly sensitive The localized surface plasmon resonance (LSPR) is the tool for localizing single plasmonic nanoparticles and collective oscillation of free electrons excited by incident studying their optical properties, local environment, and light (see Figure 1A) [1, 2]. The LSPR depends on the shape reactivity. In this review, we discuss approaches taken for [3–6], size [7–10], composition [11–13], and local envi- collecting the scattered light from single particles, their ronmentofthenanoparticle[14,15].Asaresult,theLSPR advantages and disadvantages, and present some recent is a sensitive probe of chemical reactions on the nano- applications. We introduce techniques for the excitation particle’ssurface[16–19], changes in the surrounding and detection of single-particle scattering such as high- refractive index [13, 20, 21], and charge [22] and energy angle dark-field excitation, total internal reflection dark- transfer [23] to nearby species. Further, the LSPR func- field excitation, scanning near-field microscopy, and tions as a nanoantenna [24, 25], focusing the energy of the interferometric scattering. We also describe methods to incident light to a nanoscopic volume, enhancing the achieve polarization-resolved excitation and detection. We catalytic activity [26–28], local heating capacity [29], then discuss different approaches for scanning, ratio- Raman scattering of molecules attached to the surface metric, snapshot, and interferometric hyperspectral [30–32], and fluorescence of nearby fluorophores [20, 33, 34]. Finally, as a result of their large scattering cross- sections, metal nanoparticles can be readily characterized Alexander Al-Zubeidi and Lauren A. McCarthy contributed equally to in a standard dark-fieldmicroscopeandlocalizedwith this work. nanometer precision [35, 36], allowing them to serve as *Corresponding author: Stephan Link, Department of Chemistry, nonbleaching labels for biological samples [37]. The Department of Electrical and Computer Engineering, Rice University, combination of the ability of the LSPR to efficiently drive 6100 Main Street, MS 60, Houston, TX 77005, USA, reactions, spectrally monitor these reactions, and localize E-mail: [email protected]. https://orcid.org/0000-0002-4781-930X them, has resulted in 30+ years of intensive research into Alexander Al-Zubeidi, Lauren A. McCarthy, Ali Rafiei-Miandashti and Thomas S. Heiderscheit, Department of Chemistry, the optical properties of metal nanoparticles, especially Rice University, 6100 Main Street, MS 60, Houston, TX 77005, those made of gold [38, 39]. USA, E-mail: [email protected] (A. Al-Zubeidi), [email protected] Tracking the LSPR on the single-particle level has (L.A.McCarthy),[email protected](A.Rafiei-Miandashti), emerged as the standard for characterizing the structure- [email protected] (T. S. Heiderscheit). https://orcid.org/0000-0001- function relationships of plasmonic nanomaterials 8124-4661 (A. Al-Zubeidi). https://orcid.org/0000-0002-9646- [40–42]. Both lithographically fabricated and especially 5333 (L. A. McCarthy). https://orcid.org/0000-0001-9838-8442 (A. Rafiei-Miandashti). https://orcid.org/0000-0003-4851-5065 chemically synthesized nanoparticles have heteroge- (T.S.Heiderscheit) neous size and shape distributions [43, 65]. Such Open Access. © 2021 Alexander Al-Zubeidi et al., published by De Gruyter. This work is licensed under the Creative Commons Attribution 4.0 International License. 1622 A. Al-Zubeidi et al.: Single-particle scattering spectroscopy easily averaged out and hence missed. While trends can be established, single-particle spectroscopy, often correlated with electron microscopy, is required to gain a complete understanding of nanoparticle properties [44]. To optically monitor single particles, several imaging and spectroscopic techniques have been developed including dark-field scattering [45, 46], extinction [47], photo- thermal imaging [48–50], scanning photoionization microscopy [51, 52], photoluminescence [53, 54], and transient absorption spectroscopy [55, 56]. Of the single- particle techniques, dark-fieldscatteringspectroscopyis arguably the most ubiquitous [40] due to its ease of implementation, high-throughput capabilities, and high spectroscopic resolution. Dark-field microscopy has become a popular research tool in the nanoscience community [40, 45, 46, 57]. Dark-field microscopy is based on the principle that the incident light is reflected, refracted, physically blocked, or confined in the near-field in a way that the only light collected by the detection optics is that scattered by the nanomaterial [40, 46, 58]. Dark-field excitation can be achieved via commercial dark-field condensers, evanes- cent wave excitation, subwavelength apertures, or normal incidence light with spatial filtering. Single- particle scattering spectroscopy can be implemented in adark-field microscope by attaching a spectrograph, filters, or an interferometer to characterize the intensity of the scattered light as a function of wavelength. Dark-field microscopy is nearly background-free and the large scattering cross-sections of plasmonic nanoparticles allow facile single-particle imaging, localization, and spectral acquisition. The unique capabilities of single- particle scattering spectroscopy have enabled recent advancements such as monitoring catalysis in real-time [59], measuring biomolecule adsorption [60], and quan- Figure 1: Principles of dark-field scattering. tifying single DNA modifications within cell nuclei [61]. (A) Schematic illustration of the LSPR. (B) Far-field dark-field Furthermore, in the absence of heterogeneity, dark-field scattering geometries: condenser-based (left) and objective-based scattering spectroscopy makes it possible to gain key in- HADF excitation (middle), and normal incidence dark-field excitation using a reflecting objective. Yellow represents the excitation light, sights into nanoparticle-driven reaction mechanisms [62] while orange indicates the scattering, collected either in the forward and polarized-light matter interactions [63, 64]. direction for condenser-based HADF excitation and normal This review focuses on several recently developed incidence dark-field excitation or the backward direction for single-particle scattering spectroscopy methods and is θ θ objective-based HADF excitation. The NAs and angles ( i and e) organized as follows: Section 2 covers the physical princi- listed are for excitation and collection, respectively. Angles are ples of light scattering by plasmonic nanoparticles. Section measured relative to the normal of the coverslip (white rectangles). fi (C) TIR-based near-field excitation geometries. (D) Schematic 3 describes several dark- eld excitation and collection illustration of aperture-based SNOM. geometries, with both near-field and far-field light excita- tion, scanning near-field optical microscopy (SNOM), nanoparticle heterogeneity makes quantitative moni- interferometric scattering techniques, and polarization- toring with ensemble spectroscopy difficult or impossible, resolved analysis. Section 4 describes spectroscopic and since contributions from a nanoparticle subpopulation imaging methods for single-particle scattering, including having potentially the most interesting properties can be those based on scanning, ratiometric, snapshot, and A. Al-Zubeidi et al.: Single-particle scattering spectroscopy 1623 interferometric detection. Section 5 covers specialized modes, the free electrons maintain collective oscillations, techniques for acquiring spectra in situ in electrochemical but in multiple antinodes around the surface of the par- cells or biological media, and dark-field spectroscopy ticle. The determination of lmax is dependent on the size of coupled with tip-based microscopy techniques. the sphere and the cross-sections are given by Equations (1–3) [1, 13]: 2π ∞ 2 Physical principles of light σ = ( ) ∑( + ) [ + ] ext 2 2l 1 Re al bl (1) k l=1 scattering by plasmonic 2π ∞ σ = ()∑( + )|()|2 + | |2 nanoparticles scat 2 2 l 1 al bl (2) k l=1 When light is incident on a metallic nanoparticle, the free and electrons of the metal are driven to oscillate 180° out of σabs = σext − σscat (3) phase with respect to the light’s instantaneous electric field (Figure 1A) [1]. Meanwhile, the nuclei of the particle lattice where k is the wavenumber of the incident light (k = 2π/λ). attract the electrons to return to their equilibrium posi- λ is the wavelength of light in the medium and σext, σscat, tions. Just
Details
-
File Typepdf
-
Upload Time-
-
Content LanguagesEnglish
-
Upload UserAnonymous/Not logged-in
-
File Pages35 Page
-
File Size-