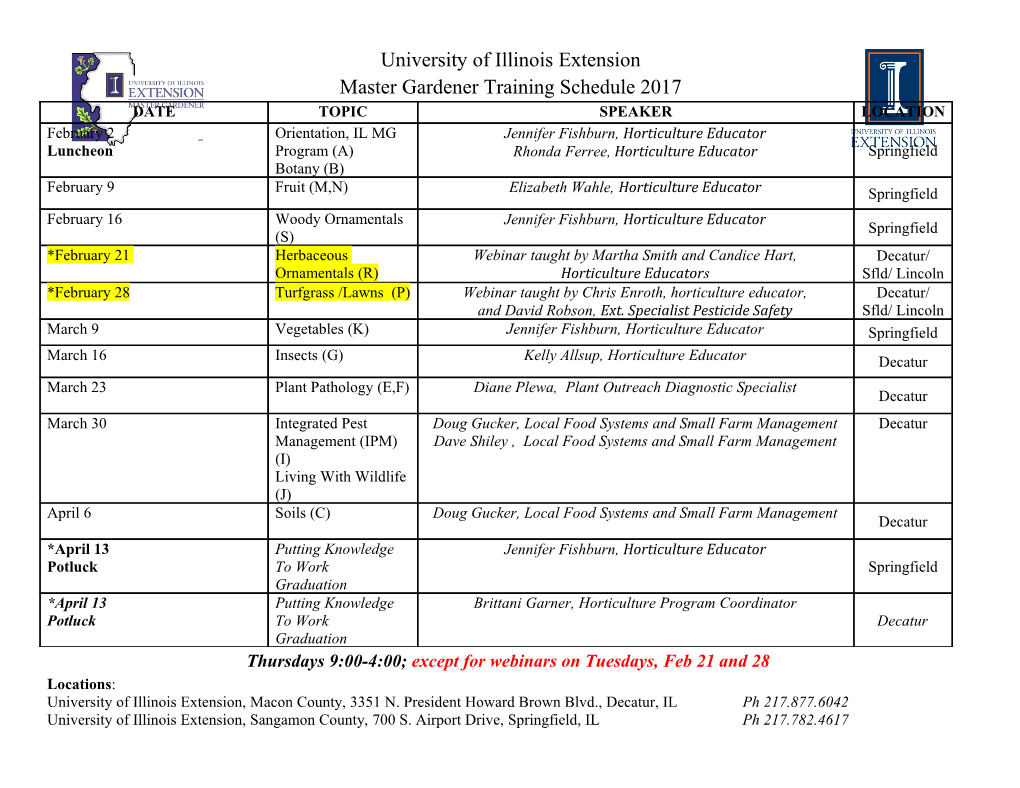
Thermodynamics of structure-forming systems Jan Korbel,1, 2 Simon David Lindner,1, 2 Rudolf Hanel,1, 2 and Stefan Thurner1, 2, 3, 4, ∗ 1Section for the Science of Complex Systems, CeMSIIS, Medical University of Vienna, Spitalgasse 23, A-1090, Vienna, Austria 2Complexity Science Hub Vienna, Josefst¨adterstrasse 39, A-1080 Vienna, Austria 3Santa Fe Institute, 1399 Hyde Park Road, Santa Fe, NM 87501, USA 4IIASA, Schlossplatz 1, A-2361 Laxenburg, Austria (Dated: January 28, 2021) Structure-forming systems are ubiquitous in nature, ranging from atoms building molecules to self- assembly of colloidal amphibolic particles. The understanding of the underlying thermodynamics of such systems remains an important problem. Here we derive the entropy for structure-forming systems that differs from Boltzmann-Gibbs entropy by a term that explicitly captures `molecule' states. For large systems and low concentrations the approach is equivalent to the grand-canonical ensemble; for small systems we find significant deviations. We derive the detailed fluctuation theorem and Crooks' work fluctuation theorem for structure-forming systems. The connection to the theory of particle self-assembly is discussed. We apply the results to several physical systems. We present the phase diagram for patchy particles described by the Kern-Frenkel potential. We show that the Curie-Weiss model with molecule structures exhibits a first-order phase transition. INTRODUCTION ied, both experimentally and theoretically, often deal- ing with particular systems, such as Janus particles [31]. Ludwig Boltzmann defined entropy as the logarithm Theoretical and computational work have explored self- of state multiplicity. The multiplicity of independent assembly under non-equilibrium conditions [32, 33]. A (but possibly interacting) systems is typically given by review can be found in [34]. multinomial factors that lead to the Boltzmann-Gibbs Here we present a canonical approach for closed sys- entropy and the exponential growth of phase space vol- tems where particles interact and form structures. The ume as a function of the degrees of freedom. In re- main idea is to start not with a grand-canonical approach cent decades much attention was given to systems with to structure forming systems but to see within a canon- long-range and co-evolving interactions that are some- ical description which terms in the entropy emerge that times referred to as complex systems [1]. Many com- play the role of the chemical potential in large systems. plex systems do not exhibit an exponential growth of A simple example for a structure-forming system, the phase space [3, 4, 8, 9]. For correlated systems it typi- magnetic coin model, was recently introduced in [15]. cally grows sub-exponentially [6{12], systems with super- There n coins are in two possible states (head and tail), exponential phase space growth were recently identified and in addition, since coins are magnetic, they can form as those capable of forming structures from its compo- a third state, i.e. any two coins might create a bond nents [9, 15]. A typical example of this kind are complex state. The phase space of thisp model, W (n), grows super- networks [16], where complex behavior may lead to en- exponentially, W (n) ∼ nn=2e2 n ∼ en log n. We first gen- semble in-equivalence [17]. The most prominent example eralize this model to arbitrary cluster sizes and to an of structure-forming systems are chemical reaction net- arbitrary number of states. We then derive the entropy works [18{20]. The usual approach to chemical reactions of the system from the corresponding log-multiplicity and { where free particles may compose molecules { is via use it to compute thermodynamic quantities, such as the grand-canonical ensemble, where particle reservoirs the Helmholtz free-energy. With respect to Boltzmann- make sure that the number of particles is conserved on Gibbs entropy there appears an additional term that average. Much attention has been given to finite-size captures the molecule states. By using stochastic ther- corrections of the chemical potential [14, 15] and non- modynamics, we obtain the appropriate second law for equilibrium thermodynamics of small chemical networks structure-forming systems. and derive the detailed fluc- arXiv:2004.06491v3 [cond-mat.stat-mech] 27 Jan 2021 [23{26]. However, for small closed systems, fluctuations tuation theorem. Under the assumption that external in particle reservoirs might become non-negligible and driving preserves micro-reversibility, i.e. detailed bal- predictions from the grand-canonical ensemble become ance of transition rates in quasi-stationary states, we inaccurate. In the context of nanotechnology and col- derive the non-equilibrium Crooks' fluctuation theorem loidal physics, the theory of self-assembly [37] gained re- for structure-forming systems. It relates the probabil- cent interest. Examples of self-assembly include lipid bi- ity distribution of the stochastic work done on a non- layers and vesicles [27], microtubules, and molecular mo- equilibrium system to thermodynamic variables, such as tors [28], amphibolic particles [29], or RNA [30]. The the partial Helmholtz free-energy, temperature, and size thermodynamics of self-assembly systems has been stud- of the initial and final cluster states. Finally, we apply 2 our results to several physical systems: We first calcu- clusters in state x(j). The mesostate is therefore char- i late the phase diagram for the case of patchy particles acterized by a vector N = n(j) , which corresponds to described by the Kern-Frenkel potential. Second, we dis- i a frequency (histogram) of microstates [? ]. The nor- cuss the fully connected Ising model where molecule for- malization condition is given by the fact that the total mation is allowed. We show that the usual second-order P (j) transition in the fully connected Ising model changes to number of particles is n, i.e., ij jni = n. For exam- N first-order. ple, a mesostate, Ψ, corresponding to a microstate Ψ (1) (2) is N = n = 2; n = 1 , denoting that there are two free particles and one two-particle cluster. RESULTS The Boltzmann entropy [35] of this mesostate is given by Entropy of structure-forming systems S (N) = log W (N) ; (1) To calculate the entropy of structure-forming sys- where W is the multiplicity of the mesostate, which is tems, we first define a set of possible microstates and the number of all distinct microstates corresponding to mesostates. Let's consider a system of n particles. Each the same mesostate. To determine the number of all single particle can attain states from the set X (1) = distinct microstates corresponding to a given mesostate, fx(1); : : : ; x(1) g. The superscript (1) indicates that the 1 m1 let us order the particles and number them from 1 to states correspond to a single particle state, and m1 de- n. By permutation of the particles we obtain the dif- notes the number of these states. A typical set of states ferent possible microstates. The number of all permuta- could be the spin of the particle f"; #g, or a set of en- tions is simply n!. However, some permutations corre- ergy levels. Having only single-particle states, the mi- spond to the same microstate and we are over-counting. crostate of the system consisting of n particles is a vec- (1) In our example with one cluster and two free particles, tor (X1;X2;:::;Xn), where Xk 2 X is the state of the permutations (4; 2; 3; 1) and (1; 3; 2; 4) correspond to k-th particle. Let us now assume that any two parti- the same microstate Ψ = (x(1); x(2)(2; 3); x(2)(2; 3); x(1)). cles can create a two-particle state. This two-particle However, permutation (2; 1; 3; 4) corresponds to the mi- state can be a molecule composed of two atoms, a clus- crostate Ψ0 = (x(2)(1; 3); x(1); x(2)(1; 3); x(1)). This mi- ter of two colloidal particles, etc. We call this state crostate is a distinct microstate corresponding to the as a cluster. This two-particle cluster can attain states (1) (2) same mesostate, NΨ ≡ NΨ0 = n = 2; n = 1 . X (2) = fx(2); : : : ; x(2) g. A microstate of a system of n 1 m2 The number of microstates giving the same mesostate particles is again a vector (X1;X2;:::;Xn), but now ei- can be expressed as the product of configurations with (1) (2) 2 ther X 2 X or X 2 X × . For instance, a state (j) k k Zn the same state for each x . Let's begin with the particles of particle k belonging to a two-particle cluster can be i that do not form clusters. The number of equivalent rep- written as X = x(2)(k ; k ). The indices in the brackets k 1 1 2 resentations for one distinct state is n(1) !, which corre- tell us that the particle k belongs to the cluster of size i two in the state x(2) and the cluster is formed by particles sponds to the number of permutations of all particles in 1 the same state. For the cluster states one can think about k1 and k2 (k1 < k2). Indeed, either k1 = k or k2 = k. Now assume that particles can also form larger clus- equivalent representations of one microstate in two steps: (j) ters up to a maximal size, m. Consider m as a fixed first permute all clusters, which gives ni ! possibili- number, m ≤ n. Generally, clusters of size j have states ties. Then permute the particles in the cluster, which (j) (j) (j) gives j! possibilities for every cluster, so that we end up X = fx1 ; : : : ; xmj g. The corresponding states of (j) j n(j) the particle are always elements from sets X × Zn with (j!) i combinations.
Details
-
File Typepdf
-
Upload Time-
-
Content LanguagesEnglish
-
Upload UserAnonymous/Not logged-in
-
File Pages19 Page
-
File Size-