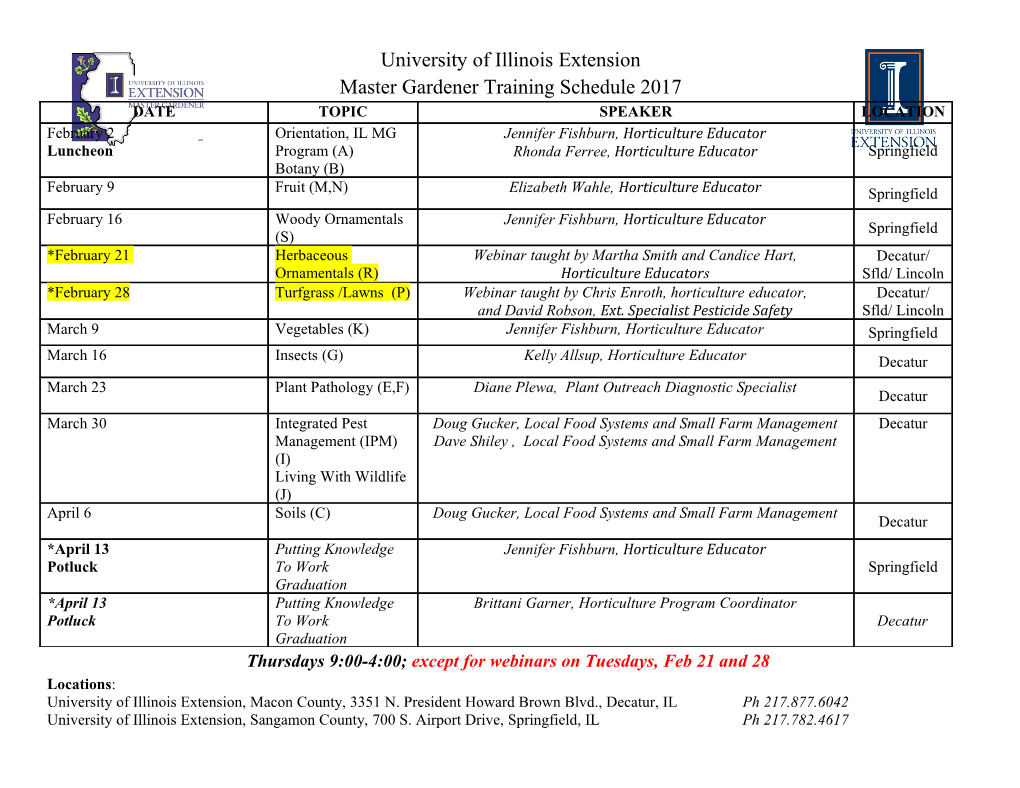
Chapter 4 / Melanocytes and Sox10 71 4 Melanocytes and the Transcription Factor Sox10 Michael Wegner CONTENTS INTRODUCTION SOX10 AND MELANOCYTES SOX10 AND MITF EXPRESSION MOLECULAR SOX10 FUNCTION CONCLUSIONS AND PERSPECTIVES REFERENCES Summary The high-mobility group (HMG) domain transcription factor Sox10 influences melanocyte develop- ment on at least two levels. Sox10 is required for survival, proliferation, and the maintenance of pluripo- tent neural crest stem cells, thereby controlling the size of the stem cell pool and indirectly influencing the number of generated melanocytes. Sox10 also directly affects melanocyte specification. Expression of Mitf, the key regulator of melanocyte development, is controlled by Sox10, which binds to and acti- vates the M promoter of the Mitf gene in close cooperation with other signaling pathways. Although its epistatic relationship to Mitf is sufficient to explain the role of Sox10 in melanocyte specification, analy- ses of expression and target genes suggest that Sox10 has additional functions in melanocytes apart from and following Mitf activation. Additionally, Sox10 is highly expressed in melanoma. It may influence melanoma properties and could therefore be of diagnostic and therapeutic value. Key Words: Sry-box; HMG; neural crest; Mitf; synergy; Pax3; Waardenburg disease; dopachrome tautomerase; tyrosinase-related protein 2; E-catenin. INTRODUCTION Transcription factors play vital roles in the development of most cell types, tissues, and organs. Sox proteins are one such group of transcription factors. They are charac- terized by possession of a common DNA-binding domain, the Sry-box (1,2) that was first identified in Sry, a protein encoded on the mammalian Y chromosome and responsible for male sex determination (3,4). This Sry-box is a variant of the HMG domain that occurs in several sequence-specific and many nonsequence-specific DNA binding pro- teins (5). In Sox proteins, it allows minor groove DNA binding in a sequence-specific manner. Because binding concomitantly introduces a strong bend into the DNA (6), Sox From: From Melanocytes to Melanoma: The Progression to Malignancy Edited by: V. J. Hearing and S. P. L. Leong © Humana Press Inc., Totowa, NJ 71 72 From Melanocytes to Melanoma Fig. 1. Topology of conserved and functional domains in the transcription factor Sox10. Positions of conserved regions 1–3 (CR1–CR3) relative to the high-mobility group domain (HMG) of Sox10 are shown. Where known, functions of conserved domains are listed. proteins are believed to fulfill architectural functions in chromatin in addition to their role as transcription factors (7). Sox proteins appear limited in their occurrence in animals, and have been detected in many different invertebrate and vertebrate species (2,8). In mammals, such as mouse and humans, 20 different Sox proteins are present. According to amino acid similarities, both within and outside their HMG domain, these 20 Sox proteins can be further subdivided into eight groups, A–H (2,8). Group E consists of Sox8, Sox9, and Sox10. In addition to the HMG domain, these proteins share three additional regions of high amino acid homology (9). One of these regions is an amino-terminal extension of the HMG domain (CR1 in Fig.1), which alters the DNA binding characteristics of group E Sox proteins, because it allows them to cooperatively bind on DNA to adjacent recognition elements (10,11). The second domain is located in the carboxy-terminal region of the protein and coincides with the transcriptional activation domain of Sox9 and Sox10 (9,12,13). Whereas clear biochemical functions were ascribed to these regions, none has yet been attributed to the third region of homology in the central part of the protein (CR2 in Fig.1). Sox10 has been identified and studied in many model organisms including human (14), mouse (15,16), rat (9), chicken (17,18), Xenopus laevis (19,20), zebrafish (21), and the puffer fish Fugu rubripes (22). Sox10 proteins are highly homologous between even distantly related species. Thus, amino acid identity of Xenopus Sox10 and mammalian Sox10 is 71% (20); and rodent and human Sox10 are 97% identical (9). In all analyzed species, Sox10 has a similar expression pattern in the developing embryo, with strong expression in the early neural crest and many of its derivatives, followed by a later phase of expression in glial cells of the central nervous system. In Xenopus, early neural crest expression appears to be furthermore dependent on Wnt and Fgf signaling and is geneti- cally downstream of snail (19,20). However, subtle differences in Sox10 expression may exist between different species. In Xenopus, for instance, Sox10 seems to be turned on sooner in cells of the premigratory neural crest than in mice (15,19,20). Although originally expressed in all neural crest cells, Sox10 is not essential for those cranial neural crest cells that form bones and mesenchyme of the face and skull. In contrast, many other neural crest cells are affected on inactivation of Sox10. The glial cells of the peripheral nervous system (23), the precursor cells for the enteric nervous system, and pigment cells (15,16,24) are completely missing in the absence of Sox10. Defects are similar in all species in which inactivations of the Sox10 gene have been observed, including humans, mice, and zebrafish. These overall phenotypic similarities notwithstanding, slight differences exist between various species. Whereas heterozygous loss of Sox10 is already sufficient in mice and men to cause partial defects of pigmen- Chapter 4 / Melanocytes and Sox10 73 tation and enteric nervous system formation (14,15), no such haploinsufficiency has been observed in the zebrafish (21). In zebrafish, Sox10 is inactivated in several allelic variants of the colorless mutant (21). In mice, a spontaneous, truncating mutation (Dominant megacolon, Dom, Sox10dom) (15,16) and a targeted mutation that removes the complete open reading frame of Sox10 (Sox10lacZ) (23) have both been reported. Additionally, SOX10 mutations were found in human patients suffering from combined Waardenburg-Hirschsprung disease (14), sometimes associated with additional central or peripheral neuropathies (25–28). Waardenburg disease is characterized by partial pigmentation defects of skin and hair combined with sensorineural deafness, whereas Hirschsprung disease results from aganglionosis of the distal colon. Sensorineural deafness in Waardenburg disease is generally believed to result from a melanocyte loss in the stria vascularis of the inner ear. However, the strong Sox10 expression in the olfactory placode and later in the olfactory epithelium is also compatible with a direct effect on inner ear epithelial cells in the case of Sox10 mutations (29). Sox10 performs multiple functions at its various sites of expression. Thus, Sox10 has been implicated in maintaining the pluripotency of neural crest stem cells (30); in pro- moting their survival and proliferation (31); in influencing fate decisions (23,32); and, at a later stage, in terminal differentiation of already specified precursor cells (33). Because Sox10 influences specification of several neural crest-derived cell lineages, it cannot exert its function alone but must cooperate with other cell-restricted signaling pathways and transcription factors that are differentially present or active in the various neural crest lineages. Thus, Sox10 is necessary for specification of peripheral glia and melanocytes, but clearly not sufficient. SOX10 AND MELANOCYTES The role of Sox10 in melanocyte development has been studied both through loss-of- function and gain-of-function analyses. Deletion of Sox10 in the mouse leads to a near complete absence of melanocytes from very early stages (15,23,24), independent of the marker that was used for melanocyte detection. Injection of Sox10 RNA into one blas- tomere of a two-cell Xenopus embryo not only led to a strong expansion of neural crest cells, but also caused these neural crest cells to preferably turn into melanocytes (19). This increase in the melanocyte pool size was only observed when ectopic Sox10 expres- sion took place before gastrulation, arguing that its effect on the melanocyte lineage is an early one (19). It has also been reported that Sox10 expression is rapidly downregulated from pigment cell precursors in zebrafish (34), again arguing that Sox10 functions early in the melanocyte lineage, either during melanocyte specification or immediately after- wards. Because Sox10 expression at this early stage is largely confined to neural crest cells and their derivatives, a cell-intrinsic function is very likely. Formal proof was obtained by phenotypic rescue of melanocytes after selective expression of ectopic Sox10 in neural crest cells from Sox10-deficient mice (35). In mammals, Sox10 expression in the melanocyte lineage appears to last longer than in zebrafish, because Sox10 can be readily detected in most melanocyte precursors migrating along the lateral pathway in mammals but not in zebrafish (15,21,23). This argues for a species-specific difference of Sox10 expression in the melanocyte lineage. Whether Sox10 expression in mammals is maintained in fully differentiated melano- cytes in vivo has not been stringently tested. However, Sox10 is strongly expressed in 74 From Melanocytes to Melanoma cultures of primary and transformed melanocytes (36–38). If its expression in mamma- lian melanocytes lasts into later stages of lineage development, Sox10 is likely to have additional effects on
Details
-
File Typepdf
-
Upload Time-
-
Content LanguagesEnglish
-
Upload UserAnonymous/Not logged-in
-
File Pages10 Page
-
File Size-