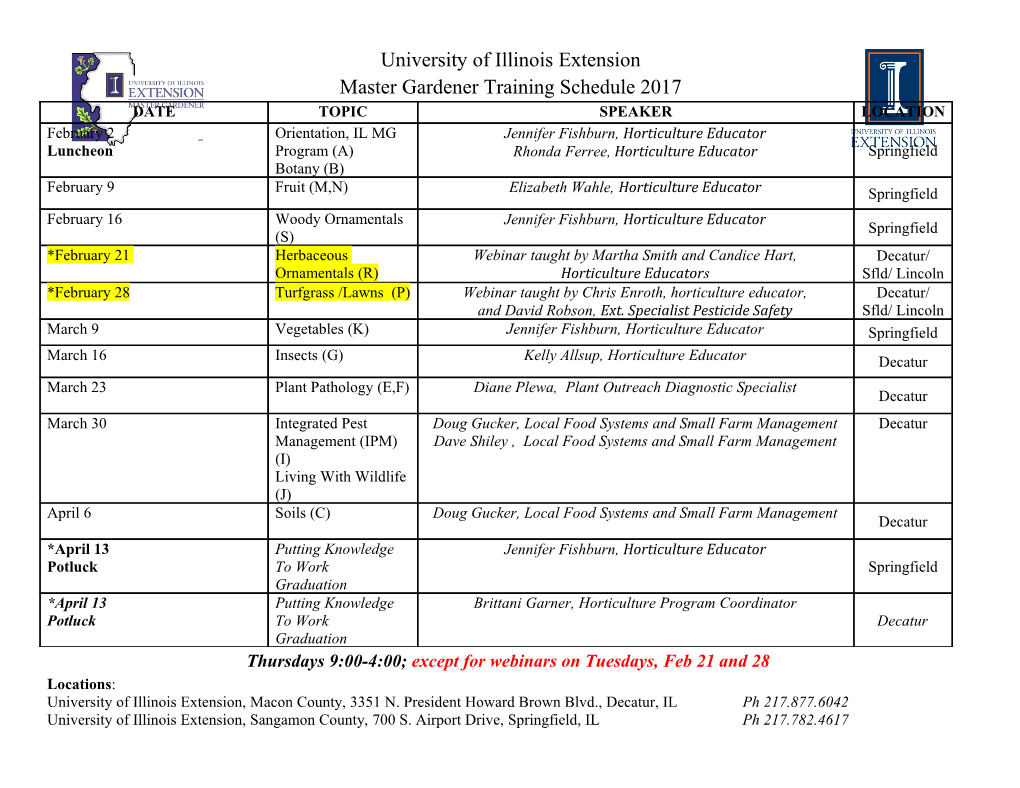
applied sciences Review Quantum Dot Solar Cells: Small Beginnings Have Large Impacts Abiseka Akash Ganesan, Arjan J. Houtepen and Ryan W. Crisp * Department of Chemical Engineering, Delft University of Technology, Van der Maasweg 9, 2629HZ Delft, The Netherlands; [email protected] (A.A.G.); [email protected] (A.J.H.) * Correspondence: [email protected]; Tel.: +31-15-27-82173 Received: 31 August 2018; Accepted: 26 September 2018; Published: 10 October 2018 Featured Application: Solution-processed optoelectronic nanomaterials for use in lighting, energy-harvesting, and sensing. Abstract: From a niche field over 30 years ago, quantum dots (QDs) have developed into viable materials for many commercial optoelectronic devices. We discuss the advancements in Pb-based QD solar cells (QDSCs) from a viewpoint of the pathways an excited state can take when relaxing back to the ground state. Systematically understanding the fundamental processes occurring in QDs has led to improvements in solar cell efficiency from ~3% to over 13% in 8 years. We compile data from ~200 articles reporting functioning QDSCs to give an overview of the current limitations in the technology. We find that the open circuit voltage limits the device efficiency and propose some strategies for overcoming this limitation. Keywords: quantum dots; lead sulfide; solar cells; photovoltaics; IV-VI semiconductors; nanocrystals; solution-processed; ligand-exchange 1. Introduction Colloidal semiconductor quantum dots (QDs) are nanometer-sized crystals made and suspended in solution. Because they can be processed in solution, have facilely tunable properties, and demonstrate unique photophysics, they are intriguing for optoelectronic devices, imaging, and fundamental science. The size and size distribution of the QD ensemble matter because they directly control the energy levels of the material (i.e., the highest occupied molecular orbital to the lowest unoccupied molecular orbital (HOMO-LUMO) gap or band gap). This observation can be explained from two points of view. The first viewpoint is a bottom-up description where the combination of molecular orbitals (energy states) with each additional atom bonded to the QD narrow the HOMO-LUMO gap until there is a negligible change, resulting in the “bulk” material. In the second top-down viewpoint, spatially confining the exciton (a bound electron-hole pair) wavefunction by making a crystal smaller thereby increases the energy required to excite the QD (e.g., the bandgap), analogous to the particle-in-a-box description [1,2]. There are several ways to synthesize colloidal QDs, which are reviewed elsewhere [3–9]. The main method, hot injection, follows a reaction pathway as described by La Mer’s model of nucleation and growth [10]. After injection, the precursor concentration in solution increases and exceeds the nucleation threshold (i.e., the solution is supersaturated); at this point, a burst of nucleation occurs and continues along with QD growth until the precursor concentration drops below the nucleation threshold and enters the growth regime. During growth, size focusing can occur if the QD growth rate is size-dependent, i.e., if smaller QDs grow faster than larger ones then the size distribution will narrow. During this time however, the high surface energy of small QDs drives their dissolution Appl. Sci. 2018, 8, 1867; doi:10.3390/app8101867 www.mdpi.com/journal/applsci Appl.Appl. Sci. Sci.2018 2018, 8,, 8 1867, x FOR PEER REVIEW 2 of2 of 28 27 precipitation onto larger QDs leading to an increase in the size distribution—A process called andOstwald’s precipitation ripening onto [5,11] larger. Influencing QDs leading these to processes an increase by in reaction the size temperature, distribution—A precursor process reactivity, called Ostwald’sand/or reaction ripening kinetics [5,11]. allows Influencing for control these processesover the size by reactionand size temperature,-distribution precursor[12–23]. Once reactivity, all the and/orreactants reaction are used kinetics and the allows concentration for control approaches over the size zero, and the size-distribution growth regime ends. [12– 23Typically,]. Once allaround the reactantshalf of the are QD’s used atoms and the reside concentration at the surface approaches [24,25] zero,. With the such growth a high regime ratio ends.of atoms Typically, exposed around at the halfsurface of the versus QD’s atomsin the residecore, atit is the not surface surprising [24,25 ].that With surface such achemistry high ratio and of atoms surface exposed environment at the surfacedominate versus many in the properties core, it is of not QDs surprising—not just that by surface size alone chemistry [26]. and surface environment dominate manyWith properties these of two QDs—not factors just in bymind size (the alone size [26 ].and the surface), this Review sheds light upon photoexcitationsWith these two in colloidal factors semiconductor in mind (the size QD films and theand surface),devices, from this absorption Review sheds to extraction light upon (and photoexcitationsthe time between). in colloidal We start semiconductor with a brief overview QD films of and the devices,processes from that absorption occur at each to extraction time scale (andranging the time from between). a few femtoseconds We start with to a briefmicroseconds overview ofbefore the processes giving an that in- occurdepth at discussion each time scaleof the rangingprogress from of Pb a few-based femtoseconds QD solar cells to microseconds (QDSCs). For before in-depth giving discussions an in-depth on discussioneach subtopic, of the we progress refer the ofinterested Pb-based readers QD solar to cells more (QDSCs). specific Forreviews in-depth [27–41 discussions]. on each subtopic, we refer the interested readers to more specific reviews [27–41]. 2. Timescales of Excited State Processes 2. Timescales of Excited State Processes In the sections below, we discuss the possible processes that occur when a QD absorbs a photon. In the sections below, we discuss the possible processes that occur when a QD absorbs a photon. A detailed understanding of each of these processes and their relevant timescales, shown in Figure A detailed understanding of each of these processes and their relevant timescales, shown in Figure1, 1, enables rational design of optoelectronic devices. We highlight various device studies that apply enables rational design of optoelectronic devices. We highlight various device studies that apply the lessons learned in each process to give an overall view of the history and state-of-the-art of Pb- the lessons learned in each process to give an overall view of the history and state-of-the-art of based QDSCs. Pb-based QDSCs. FigureFigure 1. 1. SchematicSchematic outlineoutline showingshowing thethe differentdifferent timescales of the processes in the the lifetime lifetime of of a aphotoexcita photoexcitation.tion. 2.1.2.1 Absorption. Absorption and and Multiple Multiple Exciton Exciton Generation Generation PropagationPropagation and and absorption absorption of of light light in in QD QD materials materials is is the the critical critical first first step step in in using using QDs QDs in in optoelectronics.optoelectronics. AsAs discussed discussed by by Leatherdale Leatherdale et et al. al. [42[42]] and and Ricard Ricard et et al. al. [43[43]],, the the absorption absorption cross cross sectionsection can can be be written written as: as: w 4 = j ( )j2 3 s 0휔 f w 2n1k1 p4a (1) nsc 2 3 3 휎 = ′ |푓(휔)| 2푛1푘1 ( 휋푎 ) (1) 푛푠푐 3 0 where w is the angular frequency of light, c is the speed of light, ns is the complex refractive index 0 ′ (nwheres = n s휔− isik thes) of angular the solvent frequency or surrounding of light, 푐 is medium the speed (s ofsubscript), light, 푛푠 is2n the1k1 complex= a is the refractive absorption index ′ coefficient(푛푠 = 푛푠 − of푘 the푠 ) of bulk the material solvent (1 or subscript), surroundinga is the medium radius of (s the subscript), particles, k2is푛1 the푘1 = extinction훼 is the coefficient,absorption andcoefficient the local of field the factor bulk materialf (w) is given (1 subscript), by: 푎 is the radius of the particles, 푘 is the extinction coefficient, and the local field factor 푓(휔) is given by: 3n0 2 f (w) = s ′ 2 (2) 0 2 3푛푠 0 2 푓(휔) =n + 2n (2) 1 ′ 2 s ′ 2 푛1 + 2푛푠 TheThe overall overall absorption absorption in in a a film film of of QDs QDs can can be be increased increased by by changing changing these these properties. properties. Increased Increased lightlight absorption absorption in in a a solar solar cell cell typically typically increases increases the the total total electrical electrical current current and and thus thus power power produced. produced. However,However, as as we we will will discuss discuss in in the the section section on on charge charge transport, transport, the the total total film film thickness thickness for for the the highest highest efficiency devices does not fully absorb the incident light. Therefore strategies to improve this Appl. Sci. 2018, 8, x FOR PEER REVIEW 3 of 27 Appl. Sci. 2018, 8, 1867 3 of 28 without increasing the film thickness were developed. Research groups have shown evidence of the positive impact of using plasmonic materials incorporated in QDSCs. By placing materials with a efficiency devices does not fully absorb the incident light. Therefore strategies to improve this without localized surface plasmon resonance (LSPR) [44] in the QDSC, the absorption, due to the higher local increasing the film thickness were developed. Research groups have shown evidence of the positive field factor, increased. Gold (Au) nano-shells [45], silver (Ag) nano-cubes [46], Au/Ag core/shell nano- impact of using plasmonic materials incorporated in QDSCs. By placing materials with a localized cubes [47], and also a combination of Au bipyramids and nano-spheres [48] have been tested in surface plasmon resonance (LSPR) [44] in the QDSC, the absorption, due to the higher local field factor, QDSCs.
Details
-
File Typepdf
-
Upload Time-
-
Content LanguagesEnglish
-
Upload UserAnonymous/Not logged-in
-
File Pages28 Page
-
File Size-