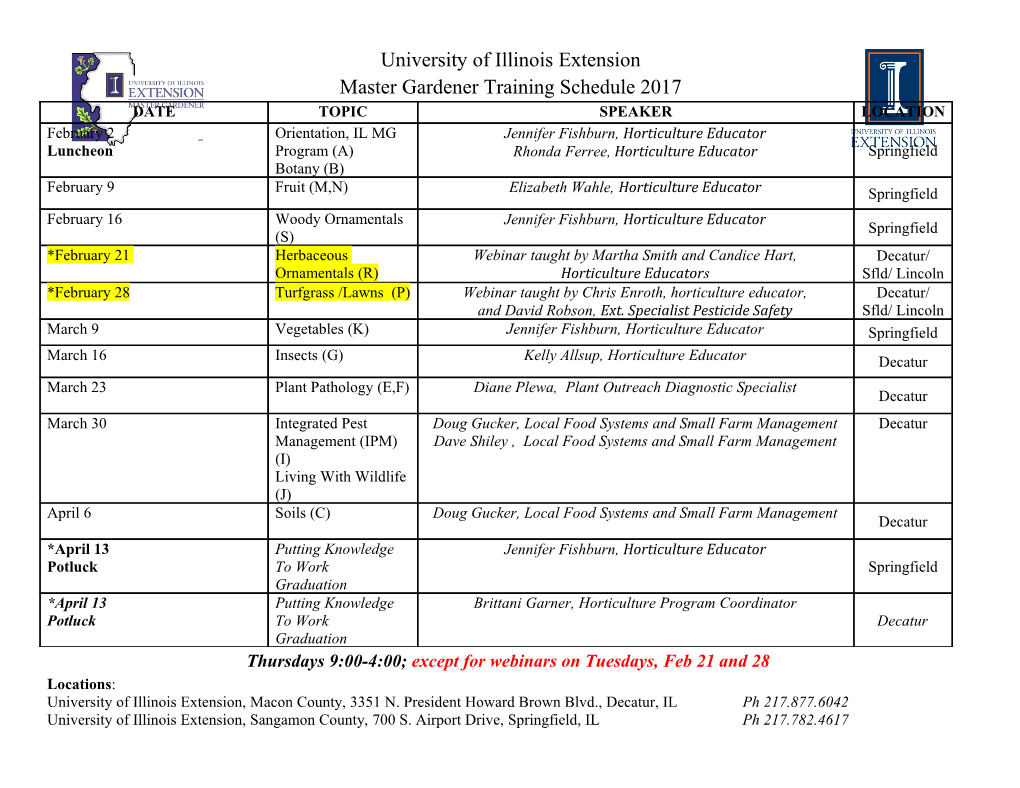
Chemical Engineering Science 153 (2016) 10–20 Contents lists available at ScienceDirect Chemical Engineering Science journal homepage: www.elsevier.com/locate/ces CuO-Fe2O3-CeO2/HZSM-5 bifunctional catalyst hydrogenated CO2 for enhanced dimethyl ether synthesis Xinhui Zhou a, Tongming Su a, Yuexiu Jiang a, Zuzeng Qin a,b,n, Hongbing Ji a,c, Zhanhu Guo b,nn a School of Chemistry and Chemical Engineering, Guangxi Key Laboratory of Petrochemical Resource Processing and Process Intensification Technology, Guangxi University, Nanning 530004, China b Integrated Composites Laboratory (ICL), Department of Chemical and Biomolecular Engineering, University of Tennessee, Knoxville, TN 37966, USA c Department of Chemical Engineering, School of Chemistry & Chemical Engineering, Sun Yat-sen University, Guangzhou 510275, China HIGHLIGHTS GRAPHICAL ABSTRACT CuO-Fe2O3 catalyst modified with different amounts of CeO2 were prepared. Addition of CeO2 led to the forma- tion of a stable Cu-O-Ce solid solu- tion. CeO2 can modify the amount of acid sites and acid type of CuO-Fe2O3 catalyst. Cu-Fe-Ce/HZSM-5 catalyst with 3.0 wt% CeO2 showed the optimal catalytic activity. article info abstract Article history: A series of CuO-Fe2O3-CeO2 catalysts with various CeO2 doping were prepared via the homogeneous Received 12 March 2016 precipitation method, characterized and mechanically mixed with HZSM-5. Their feasibility and per- Received in revised form formance for the synthesis of dimethyl ether (DME) via CO2 hydrogenation in a one-step process were 30 June 2016 evaluated. The formed stable solid solution after the CuO-Fe2O3 catalyst modified with CeO2 promoted Accepted 4 July 2016 the CuO dispersion, reduced the CuO crystallite size, decreased the reduction temperature of highly Available online 5 July 2016 dispersed CuO, modified the specific surface area of the CuO-Fe2O3-CeO2 catalyst, and improved the Keywords: catalytic activity of the CuO-Fe2O3-CeO2 catalyst. The addition of CeO2 to CuO-Fe2O3 catalyst increased Carbon dioxide hydrogenation the amount of Lewis acid sites and Brønsted acid sites, and enhanced the acid intensity of the weak acid Dimethyl ether synthesis sites, which in turn promoted the catalytic performance of CO hydrogenation to DME. The optimal Mixed oxide 2 introduced amount of Ce in the catalyst was determined to be 3.0 wt%. The CO conversion and DME Cu-Fe-Ce catalysts 2 Cerium oxide selectivity were 20.9%, and 63.1%, respectively, when the CO2 hydrogenation to DME was carried out at À1 À1 260 °C, and 3.0 MPa with a gaseous hourly space velocity of 1500 mL gcat h . & 2016 Elsevier Ltd. All rights reserved. 1. Introduction Dimethyl ether (DME) possesses a high cetane number and n produces less NO and SO than do fossil fuels when combusted, Corresponding author at: School of Chemistry and Chemical Engineering, x x Guangxi Key Laboratory of Petrochemical Resource Processing and Process In- representing an environmental friendly renewable fuel (Frusteri tensification Technology, Guangxi University, Nanning 530004, China. et al., 2015; García-Trenco and Martínez, 2012; Rutkowska et al., nn Corresponding author. 2015). DME can also be used as a chemical intermediate for E-mail addresses: [email protected] (Z. Qin), [email protected] (Z. Guo). http://dx.doi.org/10.1016/j.ces.2016.07.007 0009-2509/& 2016 Elsevier Ltd. All rights reserved. X. Zhou et al. / Chemical Engineering Science 153 (2016) 10–20 11 dimethyl sulphate, methyl acetate and low carbon olefin synthesis (XRD) to determine the crystal structure of catalysts, Raman (Ge et al., 1998). The fossil fuel usage has increased the amount of spectroscopy for obtaining the vibrational and rotation mode of CO2 in the atmosphere and the subsequent greenhouse effect the lattice and the molecules of catalysts, high-resolution trans- poses a threat to the environment. In addition, the energy crisis mission electron microscopy (HRTEM) to obtain the information facing the whole world is becoming increasingly urgent. Therefore, on the structure and composition of the particles, N2 adsorption- converting CO2 into useful chemicals, such as DME, is an attractive desorption to determine the pore structures and the texture method to reduce greenhouse-gas emissions and to recycle properties of the catalysts, H2-temperature programmed reduction carbon. (H2-TPR) to obtain the quantitative reduction information, thermal The hydrogenation of CO2 to form DME mainly includes three analysis (TG-DTA) for determining the weight changes in the cal- reactions, i.e., methanol synthesis reaction (Eq. (1)), methanol cination process of the catalysts, Fourier transform infrared spec- dehydration reaction to form DME (Eq. (2)) and a reversed water- troscopy (FTIR) of adsorbed pyridine and temperature-pro- gas shift reaction (Eq. (3))(Bonura et al., 2014b; Yang Lim et al., grammed desorption of ammonia (NH3-TPD) for obtaining the 2016). DME can be produced from CO2 via two routes. The first surface acidities of the catalysts, and temperature programmed route is a two-step process through two reactors (including me- oxidation (TPO) to detect the coke formed during the reaction. The thanol synthesis on a metallic catalyst and subsequent dehydra- catalysts were mixed with HZSM-5 to synthesize dimethyl ether tion of methanol on an acid catalyst). The second route is a one- via CO2 hydrogenation in a one-step process. step process using a bifunctional catalyst in the same reactor to simultaneously perform the two steps. However, methanol dehy- dration in the same reactor disturbs the equilibrium of synthesis 2. Experimental reaction. Therefore, one-step process is economically and ther- modynamically favored (Jia et al., 2006; Vakili et al., 2011). 2.1. Catalyst preparation À1 CO2 þ3H2⇋CH3OHþH2O; ΔH298¼49.46 kJ mol (1) The precursor for producing the CuO-Fe2O3-CeO2 catalyst was prepared via a homogeneous precipitation method. ⇋ þ Δ ¼ À1 2CH3OH CH3OCH3 H2O; H298 23.5 kJ mol (2) Cu(NO3)2 Á 3H2O and Fe(NO3)3 Á 9H2O (Sinopharm Chemical Re- agent Co., Ltd.) were weighed at Cu/Fe mole ratio of 3:2 (Liu et al., À1 CO2 þH2⇋COþH2O; ΔH298 ¼41.17 kJ mol (3) 2013; Qin et al., 2015; Qin et al., 2016). Then, the amount of the Ce(NO3)3 Á 6H2O (Sinopharm Chemical Reagent Co., Ltd) was de- The bifunctional catalysts for the one-step synthesis of DME via termined by the desired CeO2 content of 1.0, 2.0, 3.0 and 4.0 wt% in CO hydrogenation include a Cu-base catalyst for the hydrogena- 2 CuO-Fe2O3-CeO2 in the final catalysts and was added to obtain a γ À1 tion component and a solid acid, such as HZSM-5, -Al2O3 or HY metal nitrate solution (0.5 mol L ). A urea (China Guangdong zeolite for the dehydration component, resulting in Guanghua Sci-Tech Co., Ltd.) solution (5 mol LÀ1) was then added CuO-ZnO-Al2O3/HZSM-5 (Liu et al., 2015), CuO-ZnO-Al2O3-ZrO2 to the metal nitrate solution, in which the urea to nitrates molar /HZSM-5 (An et al., 2008), Cu-ZnO-ZrO2/HZSM-5 (Frusteri et al., ratio was 20. The mixture was stirred and reacted for 24 h at 90 °C. 2015), CuO-TiO2-ZrO2/HZSM-5 (Wang et al., 2009)or The obtained precipitates were filtered and dried at 110 °C for 12 h, CuO-Fe2O3-ZrO2/HZSM-5 (Liu et al., 2013; Qin et al., 2015). How- ground through a 20–40 mesh, calcined at 400 °C for 4 h, and thus ever, the conversion of stable CO2 molecule requires high tem- the catalyst CuO-Fe2O3-CeO2 was obtained for methanol synthesis. perature and pressure and only 10–30% was reported with the HZSM-5, using as the methanol dehydration component, with a DME selectivity of only 30–50% when the reaction was carried out silica-alumina ratio of 300:1 (China Shanghai Novel Chemical – – ° at 4 10 MPa and 200 300 C. Due to the conversion of CO2 to Technology Co., Ltd.) was mechanically mixed with the methanol was limited by the thermodynamic equilibrium, it was CuO-Fe2O3-CeO2 oxide composites at a mass ratio of 1:1. For fi more dif cult to attain reasonable per-pass conversions for large comparison, a CuO-Fe2O3/HZSM-5 catalyst without CeO2 was industrial scale CO2 treatment. prepared via the same method. CeO2 is an effective co-catalyst with a hollow structure, and can stabilize and disperse Cu and other precious metals (Bera et al., 2.2. Catalyst characterization 2003). CeO2 and CuO can form a stable solid solution (Gamarra et al., 2007; Marbán and Fuertes, 2005) to improve both catalytic The XRD, N2 adsorption-desorption and H2-TPR catalyst char- activity and thermal stability of CuO-based catalysts. CeO2 has acterizations can be referenced in previous studies (Liu et al., been frequently selected as a component for use in complex oxides 2013). Briefly, the X-ray diffraction (XRD) analysis was performed or as a dopant to improve the catalyst performance. CeO2 has been using a Bruker D8 Advance X-ray diffractometer. The isotherm of applied in the field of preferential CO oxidation (Hornés et al., nitrogen adsorption and desorption was measured by an ASAP 2010; Jia et al., 2010) and methanol steam reforming (Tonelli et al., 2000 physical adsorption instrument (Micromeritics Instrument 2011). For the dimethyl ether synthesis from CO2, the acid prop- Corporation). The catalyst surface area was calculated via Bru- erties of the catalysts influenced the DME selectivity (García- nauer-Emmett-Teller (BET) method, and the pore size distribution Trenco and Martínez, 2012; Yoo et al., 2007). For example, the curve was determined using the Barrett-Joyner-Halenda (BJH) acidity of zeolite HY was enhanced by the addition of Ce, and it model, which was based on the isotherm of desorption side.
Details
-
File Typepdf
-
Upload Time-
-
Content LanguagesEnglish
-
Upload UserAnonymous/Not logged-in
-
File Pages11 Page
-
File Size-