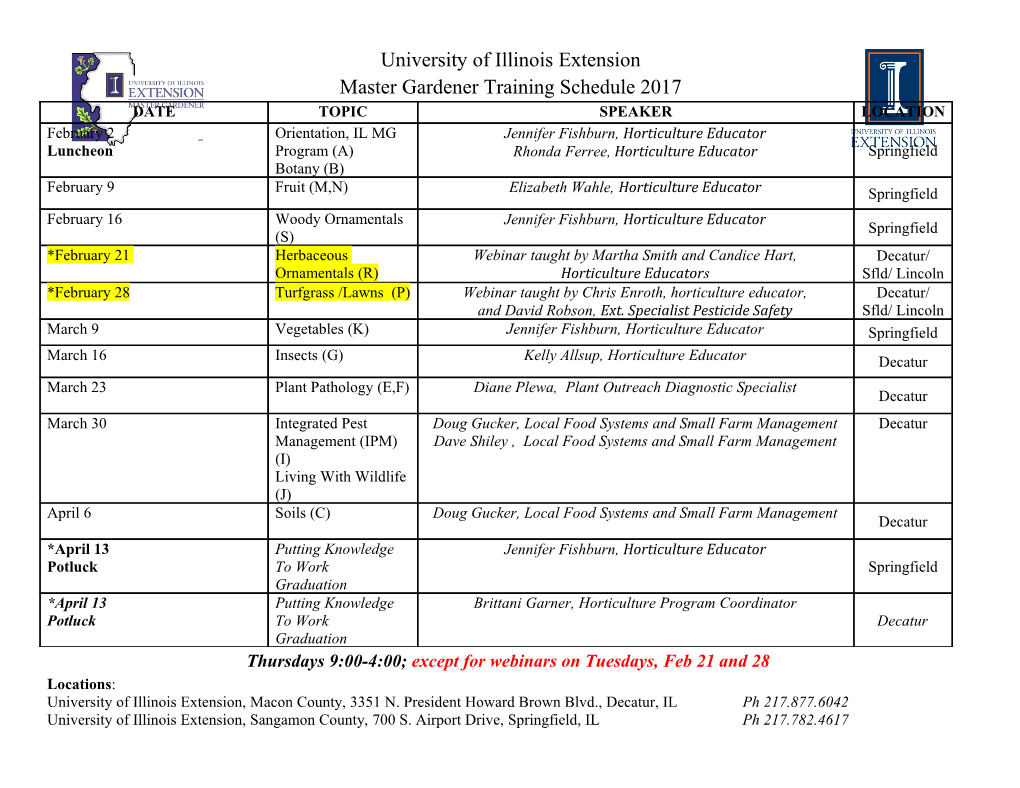
Institute for Chemical and Bioengineering Department of Chemistry and Applied Biosciences ETH Zurich 8093 Zurich,Switzerland LECTURE NOTES (intended to complete but not to substitute the lectures) POLYMER REACTION & COLLOID ENGINEERING Prof.Dr. M. Morbidelli Prof.Dr. P. Arosio November 17, 2016 AUTUMN SEMESTER 2016 Contents 1 Free-Radical Polymerization 6 1.1 Chemical Reactions . 6 1.1.1 Initiation . 6 1.1.2 Propagation . 7 1.1.3 Chain Transfer . 7 1.1.4 Bimolecular Termination . 9 1.2 Diffusion Control of Chemical Reactions . 9 1.3 Polymerization Processes . 11 1.3.1 Bulk Polymerization . 11 1.3.2 Solution Polymerization . 11 1.3.3 Suspension Polymerization . 11 1.3.4 Emulsion Polymerization . 11 1.4 Kinetics of Free-Radical Polymerization . 12 1.4.1 Involved Chemical Reactions . 12 1.4.2 Population Balance Equations in a Batch Reactor . 12 1.4.3 Rate of Monomer Consumption . 14 1.5 Pseudo Steady State Approximation . 15 1.5.1 Stiffness Ratio . 18 2 Chain Length Distribution of Polymers 20 2.1 Population Balance Equations . 20 2.1.1 Active Chains . 20 2.1.2 Dead Chains . 22 2.2 Instantaneous Chain Length Distribution . 24 2.2.1 Number Distribution and Number Average . 24 2.2.2 Weight Distribution and Weight Average . 25 2.2.3 Polydispersity . 26 2.2.4 Summary . 27 3 Cumulative Properties of Polymers 28 3.1 Cumulative Chain Length Distribution, Moments, and Properties . 28 3.1.1 Width of the Cumulative Distribution . 30 3.2 Designing Polymerization Processes with Respect to Desired CLDs . 31 3.2.1 Case I: Termination by Combination is Negligible . 31 2 Contents 3.2.2 Case II: Termination by Combination Dominates . 33 4 Copolymerization 35 4.1 Kinetics of Copolymerization . 35 4.1.1 Involved Chemical Reactions . 35 4.1.2 Long-Chain Approximation . 36 4.1.3 Rate of Monomer Consumption . 37 4.2 Chain Length Distribution of Copolymers . 38 4.2.1 Population Balance Equation . 38 4.2.2 Pseudo-Homogeneous Approach . 38 4.2.3 Kinetics of Multi-Monomer Polymerization . 39 4.3 Chain Composition Distribution of Copolymers . 41 4.3.1 Instantaneous Chain Composition . 41 4.3.2 Composition Drift . 43 4.3.3 Chain Composition Control . 44 4.3.4 Reactor Monitoring and Control . 50 5 Heterogeneous Free-Radical Polymerization 53 5.1 Concept of Micellar Particle Nucleation . 53 5.2 Radical Segregation . 54 5.3 Phase Partitioning . 56 5.3.1 Monomer . 57 5.3.2 Emulsifier . 57 5.4 Colloidal Stability . 58 5.5 Kinetic Mechanism of Emulsion Polymerization (Smith & Ewart, 1954) . 58 5.6 Kinetics of Micellar Particle Nucleation . 61 5.7 Distribution of Active Radicals in the Polymer Particles . 63 6 Stability of Colloidal Suspensions 67 6.1 Kinetic Stability of Colloidal Suspensions . 67 6.2 Interaction between two Charged Bodies . 68 6.2.1 van der Waals Forces . 68 6.2.2 Electrostatic Forces . 72 6.2.3 Total Interaction Energy . 84 6.2.4 Simple Manifestation of Electrical Double Layers: Soap Films . 85 6.3 Coagulation of Colloidal Suspensions . 86 6.3.1 Aggregation by Potential Control . 86 6.3.2 Aggregation by Electrolyte Addition . 88 6.4 Steric Interactions . 91 3 Contents 7 Kinetics and Structure of Colloidal Aggregates 95 7.1 Diffusion Limited Cluster Aggregation – DLCA . 95 7.1.1 Aggregation Rate Constant – DLCA . 95 7.1.2 Cluster Mass Distribution – DLCA . 97 7.1.3 Role of Aggregate Morphology – DLCA . 98 7.2 Reaction Limited Cluster Aggregation – RLCA . 100 7.2.1 Aggregation Rate Constant – RLCA . 100 7.2.2 Role of Cluster Morphology – RLCA . 103 7.2.3 Cluster Mass Distribution – RLCA . 105 7.3 Comparison of Aggregation Regimes – DLCA vs. RLCA . 105 7.3.1 Aggregation Rate Constant – DLCA vs. RLCA . 105 7.3.2 Cluster Mass Distribution – DLCA vs. RLCA . 106 7.3.3 Aggregate Morphology – DLCA vs. RLCA . 109 7.3.4 Comparison to Experimental Data . 110 7.4 Cluster Coalescence . 111 7.5 Solid Suspensions under Shear . 116 7.5.1 Shear induced Aggregation . 116 7.5.2 Shear induced Breakage . 121 7.6 Gelation of Colloidal Suspensions . 122 7.6.1 The Gelation Process . 122 7.6.2 Brownian-Induced Gelation . 124 7.6.3 Shear-Induced Gelation . 125 7.7 Experimental Characterization of Colloidal Suspensions . 128 7.7.1 Light Scattering . 128 7.7.2 Static Light Scattering . 129 7.7.3 Dynamic Light Scattering . 135 7.8 Zeta Potential, Electrophoretic Mobility, and Surface Charge Density . 137 8 Proteins and Bioprocesses 142 8.1 Proteins and Biomolecules . 142 8.2 Enzyme Kinetics . 146 8.2.1 Michaelis-Menten Equation . 146 8.2.2 Engineering of enzyme kinetics . 147 8.3 Bioprocessing . 149 8.3.1 Microbial growth . 149 8.3.2 Reactor design . 150 8.3.3 Product recovery, formulation and delivery . 153 8.3.4 Choice of the reactor configuration . 153 8.3.5 Biopharmaceuticals . 154 9 Protein Aggregation 162 9.1 Proteins and Colloids . 162 4 Contents 9.2 Experimental biophysical techniques . 164 9.3 Kinetic Model and PBE . 167 9.3.1 Case Study: aggregation of a monoclonal antibody (mAb-1) . 168 9.4 Protein Misfolding and Aggregation in the Biomedical Context . 173 9.4.1 Functional role of protein self-assembly and aggregation in biology . 173 9.4.2 Role of aberrant protein aggregation in pathology: amyloid fibrils . 174 9.4.3 Kinetic and thermodynamic stability of amyloid fibrils . 175 9.4.4 Aggregation mechanism of amyloid fibrils . 177 9.4.5 Role of chemical kinetics in drug discovery . 178 5 Chapter 1 Free-Radical Polymerization 1.1 Chemical Reactions 1.1.1 Initiation The initiation reaction produces free radicals. There are several ways to do this: • Chemical initiation The decomposition of the initiator (e.g. AIBN) forms free radicals: CH3 CH3 CH3 kd NCCN N C CN N2 + 2 NC C CH3 CH3 CH3 kd • • I2 −! I + I (rd = kdI2) • kI • I + M −! R1 dI• = 2 f k I − k I• M ≈ 0 (1.1) dt d 2 I • ) kII M = 2 f kdI2 ≡ RI (1.2) where f is the initiator efficiency, typically f = [0:5; 1]. Note that in order to ensure a continuous production of radicals all over the process, 1=kd should be larger than the characteristic time of the polymerization reaction. Examples of the decomposition characteristic time, τd for some commercial initiators are: 6 CHAPTER 1. FREE-RADICAL POLYMERIZATION τd T Acetyl peroxide 2 h 80 ◦C Cumyl peroxide 12 h 110 ◦C t-Butyl hydroperoxide 45 h 150 ◦C Since this is a first order process, τd = 1=kd. • Thermal initiation: thermal decomposition of the monomer (e.g. styrene). This represents a danger, for example during monomer transportation, since it may lead to undesired polymerization of the monomer. For this reason, inhibitors (scavengers of radicals) are usually added to the monomers before storage. This causes the occurrence of a non reproducible induction period when such monomers are polymerized. • Initiation by radiation The decomposition of the initiator is caused by light or another source of radiation. Since this method is quite expensive, it is only applied to polymerization systems oper- ating at very low temperatures. 1.1.2 Propagation Propagation is the addition of a monomer molecule to a radical chain. R R k + p n-1 n k • p • • Rn + M −! Rn+1 rp = kpRn M 1.1.3 Chain Transfer • Chain transfer to monomer • kfm • • Rn + M −! Pn + R1 r = kfmRn M The reactants are the same as for the propagation reaction, but the activation energy is 3 much larger. Accordingly, kfm is usually at least 10 times smaller than kp. This reaction 7 CHAPTER 1. FREE-RADICAL POLYMERIZATION R R k + fm + n-1 n-1 leads to the formation of a polymer chain with a terminal double bond. This can induce chain branching through the terminal double bond propagation reaction. • Chain transfer to chain transfer agent • kfs • • Rn + S −! Pn + R1 r = kfsRnS A chain transfer agent, S is a molecule containing a weak bond that can be broken to lead to radical transfer, similarly as in the case of monomer above (e.g. CCl4, CBr4, mercaptans). • Chain transfer to polymer k • fp • • Rn + Pm −! Pn + Rm r = kfpRn (mPm) • In this reaction the growing radical chain, Rn extracts a hydrogen from the dead chain, Pm. Since this extraction can occur on any of the m monomer units along the chain, the rate of this reaction is proportional to the length of Pm. General observations on the role of chain transfer reactions: - The concentration of radicals is not affected and therefore the rate of monomer con- sumption is also unchanged. - The growth of polymer chains is stopped and therefore shorter chains are produced. - Each transfer event leaves a different end-group on the chain that can be detected (NMR, titration) so as to identify and quantify the corresponding chain transfer reaction. - Nonlinear (branched) polymer chains are produced: directly by chain transfer to.
Details
-
File Typepdf
-
Upload Time-
-
Content LanguagesEnglish
-
Upload UserAnonymous/Not logged-in
-
File Pages182 Page
-
File Size-