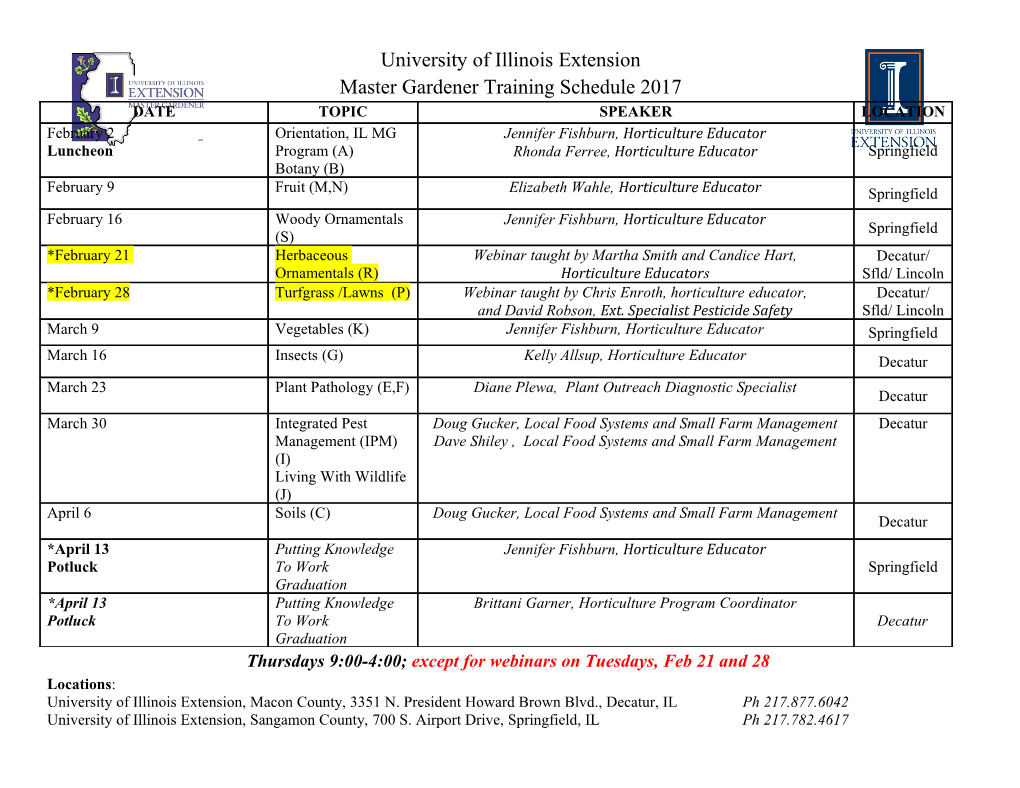
BASIC RF THEORY, WAVEGUIDES AND CAVITIES G. Déme CERN, Geneva, Switzerland ABSTRACT After a brief mathematical introduction, Maxwell's equations are discussed in their most general form as known today. Plane waves are considered in unbounded media, with the laws of reflection and refraction at a planar interface. A general theory of waveguides and cavities is then developed in detail. Periodically loaded waveguides are given as an example of accelerating structure. The parameters which characterize the interaction of resonant cavities with a particle beam are introduced, for both longitudinal and transverse motions. 1 . MATHEMATIC AL REPRESENTATION OF PHYSICAL VARIABLES AS A FUNCTION OF TIME 1 . 1 Sinusoidal variables (time-harmonic electromagnetic fields) Phasors Real sinusoidal variables a(t) = am cos (cot + tp) are represented as Rea¤’(°"*°)=l,,,] ReA[u] cf (1.1) where A el"' (with to 2 0) is called a phasor and A = dm ef'? is its complex amplitude. When the differential equations determining the physical variable a(t) are linear with real coefficients, any complex solution of the type A elm yields two real solutions which represent physical quantities: Re(A ew)l = |A| cos (mz + q>) Irn(A e)lw = |A| sin (mz + rp) Since these solutions merely differ by the time origin, it is sufficient to keep only the first one. A (capital letter) is the complex amplitude of the real sinusoidal variable a(z) written as a lower case letter. IAI is the amplitude am; arg (A) = o is the phase of a(t). In Eq. (1.1), the phasor A el"' may be replaced by its complex conjugate; in fact both conventions are used in the literature. Here we will adhere to the elw convention because it is always used in RF engineering, in particular in all measuring instruments; the e·i°" convention is often used in theoretical pyromhsics. one conventionIn order to topy p_ytranslate (e usefulness results of phasors f stems from the fact that they theare eigenfunctions other, simlrelaceof the operator [dt, jb-i). with eigenvalue Th jo). The exponential eJ°" can be factorized out of all linear differential equations; f`mally the equations are written using the complex amplitudes of all variables, with 8/32 replaced by jco. This formalism also applies to sinusoidal variables which are exponentially damped with time. In that case to is complex: jm = jwl — otl where co1,ot] are real positive quantities (1.2) OCR Output le .=2 lR(lEoj e. =l2` Re(e) 2 2* 4 ·2|2 OCR OutputVector (or cross) product [5.;;*] = EXH; - EyH; 7 (1.5) 1 -· -• * . .. Time ··averae g[l of e >< h= Re5[] E x H (1-6) C·0l'l’\§l€X vector 1 . 2 General time variation Fourier vamforms For a general time variation, we use Fourier transforms: 1 im +°° (1.7) - Jwt -dn —j¤¤¢ *00 f(z)-T jF(m) e dw F(co)- jf(z) e dt This representation uses positive and negative frequencies, i.e. a two-sided frequency spectrum. If j(t) is real, F (-0)) = F *(c0) (1) and f(t)=Re i [ F ( to )e"°‘ dco (1-8) Complex representation of the variable f(t), as a superposition of phasors with positive frequencies (one-sided Bequency spectrum). Laplace transforms Convergence condition: With to real, in (1.7) y‘(t)| must decrease fast enough when z -> :i: To get around this problem, we use unilateral Fourier transforms: F+(m) = jf(t) e`J°°'dz F_(co) = _[f(t) e`}°xdt (1-9) With p = c +j0), F+#=p °° [)J lf(*) 0 ==""d* F-rN0)p ° K} J -... ¤""d* (*-10) OCR Output F .,. may be continued analytically for c 2 a where a S 0 F - may be continued analytically for c S b where 0 S b Let H(t) be Heaviside's unit step ftmction: H(t)-·=O I/2for t <for 0 1 for l=() t > O F +(t0) is the Fourier transform of the causal function j(t) H(z); F +(p/ J) is its Laplace transform. (1) This relation holds for 0) real; for to complex we would have F (-0)) = F *(0J*). Inversion formula for Laplace tran.sfonn.· f(t)H(t)=-jF+;ep'dp1 c+j¤¤ 5;} t (£j) C··_]°° as c f(¢)H(—r)1 c+j¤¤= ?]+ 2mI C- ,.1.F.<="‘dp 1 C S b f(t)=—jc+j¤=· L 21¤1 C- ,.. 1eP‘dp ascsb (1.11) Hilbert trartsfomts The Hilbert transform of a complex f1mctionf(x) defmed on the real axis is another complex function g(y) also defmed on the real axis by %lf(x)]= 8(>’)=%lt:%%dx (1.12) where the integral is taken as a Cauchy principal value. It can be shown that l"°° gw — l— H[g<x>] —— -.- ——·1x - — fm 113 < . > rc x — y Examples 1) Hilbert transform of elw with respect to t . ,. %t¢’°"= [] $21¤>¢$i% rdt =. c"l1:%dt, 1<¤¢· = .iefli:?@dt , ·°' `w =j Q sgn(m) el‘°‘ (1.14) 2) Hilbert transform of eJ°°·’ with respect to 0J 1¤>¢ H,,,e’°°’=lf;-;d0a=j. []lLTC co - co sgn(t) w. u (1.15) Causal fimction in time: f(t) = 0 for t < O. Its Fourier transform then reads F(w) = [ f (t) e"‘°’dt With (1.15) ¤t[F<<»>1 = iii: —@Tr<¤> elect = To wilt: &¤—1“ = -11d* rw ¤. ,"°"’ TC (D " (D0 0 K (D — (D 3·[[Re F(m)]=rm Fw) . , H,[F(w)] = —] F(0J ) hence Hum F(w)]=_Rc Fm,) (1.16) OCR Output Causal function in frequency: F (ca) = 0 for on < 0. Its Fotuier transform reads °j‘°' f(z)= $f;F(w)E edc0 With (1.14) 1 dz 1 ’ 1 °° 1 ,, dt · j °° ... —· it =—;,,—-F,-i2.,, me =—d F -ig-f°"=—dzi,-,21t) F J Wl J »{(wl °°’ g °’ (WJ ·° g°’ "°’° _ . , at[R¤f(i)]=-1mf(»·) 3·£[f(t)] — j f(t ) hence H[Imf(!)]=Rcf(t,) (1.17) Application: In (1.8) we write jF(w) e’°"dw = f(z)+ jg(z) = A(¢) em=') s(t) with f,g and A, tp real (1.18) The complex function s(t) is of the type f(t) in (1.17); therefore a£[f(:)] = -g(i’) ic. go') = -%l;;%%¤1i“£ (1.19) rpartTo the isreal obtained functiongt) as [f(z)]. we can associate a complex signal s(z) whose real part is f(t), and whose imaginary The modulus and argument of s(z) allow a natural defmition of the instantaneous amplitude, phase and frequency of the real function j(t) as [1, 2, 3, 4] d r ) Am = Ist:) <t><¢> = arg [sm] no) (1-20) Moreover +¤¤2 ju): +¤¤-icl [ locA (z)dz= I dz;fF(m)1 ecc dmtjF * [(co) _y! e dm —X —00 0 2°°°° 2°° 2*+3 2+°°2 R · * ·· -F ‘2()d gdmgdw F(u>)F (to) 5(m—w )=ijdu>|F(m)] -;j c¤| (m)| - { f z z from Parseval's formula applied to (1.7). Therefore, since A2(t) =j2(t) + g2(t), "2+°2`°2 Fr<»>¤»=fg<¤>¤¢=§fA<¢>¤¤ wv OCR Output This relation is similar to (1.4b). 1 .3 Useful vector identities We will make repeated use of the following vector identities: z1·[Bxa]=5·[a¤a]=s·[ax5] [a><[5xa]]=(a-e)5-(5-5)e [z1><5]·[z><&`]=(a-s)(5-J)-(a·J)(5-e) div(U5)=E1’·gradU+ U div& div(U gradV)=gradU~gradV+U AV C11I’l(U§)=[gI3dUX5]+ U curlii curlgradV =0 d1v[axt`5]=5-¤¤i1a- zi-e¤r115 dnvcuina :0 2 . MAXWELL'S EQUATIONS AND SOME APPLICATIONS 2 . 1 Maxwell's equations J = free electric current density p = free electric charge density 1,,, = free magnetic current density pm = free magnetic charge density Although magnetic currents and charges do not exist in nature, they are useful mathematical tools as equivalent sources for electromagnetic fields ([5]. P. 46 and 52). curlFI=]+°%? divD=p (2.1) em1E=-i d1vB=p,,, m gf Since div curl = O, dnvhzo (2.2) g anvi,+%??=o,, Equations (2.2) express the conservation of electric and magnetic charges. C onsrizuzive relaiions These relations link the inductiorns D, E to the fields E, I? ; they describe tlne electromagrnetic properties of a medium. In general D = e0E+° F B=. ucl? + p.0M (2-3) where P = electric polarization = electric dipole moment density u0M = magrnetic polarization = magnetic dipole moment density M = magnetisation (equivalent electric current == curl M) The definition (2.3) introduces an asymmetry between I3 and M. This is an historical definition ([6],_p. 12) and the most common one in the literature; but some authors do not write a factor No in front of M. At a given time, P. and M depend on the previous history of the sample. For linear media, by superposition P(t) can be written as a convolution OCR Output - f .. +¤· .. P(t) = jp(z — t') E (z')dz'= fp(z — z') E (z')d:’ (2.4) where p(t)=0 for t <0 . which is the definition of a causal fxmction lll time. In frequency domain with 01 real, (2.4) becomes (here the Fourier transforms are represented by the same symbols as the time variables) P(<·>> = p(w) E (w) with p(—<¤> = p`<<¤) so that D(0>) = e(w) E (co) where e(m)= so + p(m) The relation between D and E is simple only in frequency domain. In general, e(c0) is complex: e(c¤)= e’(<n)— je"(m) with c(—m)= c"(m) z c' even, c" odd function of oi (2.6) Since p(t) is a causal function, from (1,13) Re p(m) = Re [s(m) - :50)] and lm p(w) = lm [s(c0)] are Hilbert transforms of each other: 4-no n • _ 4-oe n 1 ·c"(w) = i { —-—-ag (°{) 80 ot €·(w) - 80 Z l { --8 ,(°°) dw (2.7) rc ___ to -0) 1t___ w -0J Since e' is an even and e" is an odd function of 0), Eq.
Details
-
File Typepdf
-
Upload Time-
-
Content LanguagesEnglish
-
Upload UserAnonymous/Not logged-in
-
File Pages96 Page
-
File Size-